STE Highlights, August 2023
Awards and Recognition
Experimental Capability Enhancement
Experimental platform leveraged for investigations at National Ignition Facility
Materials Physics and Applications
‘Goldilocks’ approach brings ‘just right’ water management to unitized reversible fuel cells
Materials Science and Technology
Quantifying additive manufacturing could help repair high-value components
Awards and Recognition
Marcelo Jaime to serve condensed matter research community on PRB editorial board
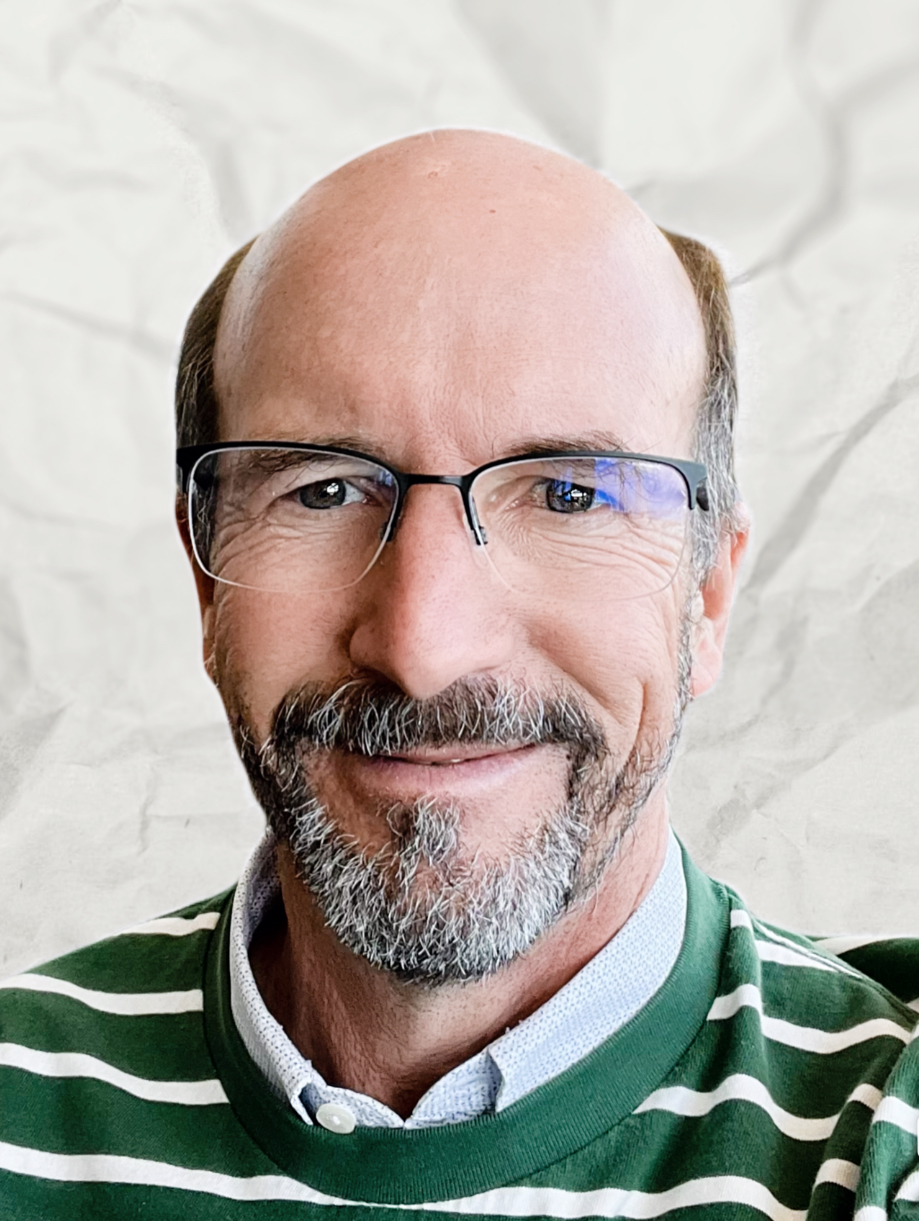
Marcelo Jaime
Marcelo Jaime (MPA-MAGLAB) has been appointed to the editorial board of Physical Review B. His three-year term began in May. As a board member, Jaime joins a diverse, international team of active, distinguished scientists selected by the journal editors to provide advice during the peer-review process.
Physical Review B is the world’s largest dedicated physics journal, publishing roughly 100 new papers each week, with authors and referees from over 130 countries. The most highly cited journal in condensed matter physics, Physical Review B is part of the Physical Review family of journals published by the American Physical Society (APS).
As a scientist at the National High Magnetic Field Laboratory-Pulsed Field Facility, Jaime has developed techniques to better understand emergent behavior of quantum materials by measuring their thermal and lattice properties in very high magnetic fields, both pulsed and continuous, at low temperatures. He joined the Lab in 1997 as a postdoctoral researcher, performing the first-ever specific heat measurement in pulsed magnetic fields up to 60 Tesla. Prior to joining the Lab, Jaime earned a doctoral degree in physics from Instituto Balseiro, in Bariloche, Argentina with a doctoral fellowship from CONICET — the national scientific and technical research council of Argentina — and completed a postdoctoral appointment at the University of Illinois Urbana-Champaign.
Jaime is a fellow of the APS and the American Association for the Advancement of Science. He is the recipient of the Tooru Atake Lectureship Award from Japan Society of Calorimetry and Thermal Analysis, the “Science above 100T” Team Distinguished Performance Award from Los Alamos National Laboratory and the James J. Christensen Memorial Award from Brigham Young University. He currently serves as past chair in the four-year chair line for the APS Topical Group on Magnetism and its Applications.
Sevanto named to Scientific Reports editorial board
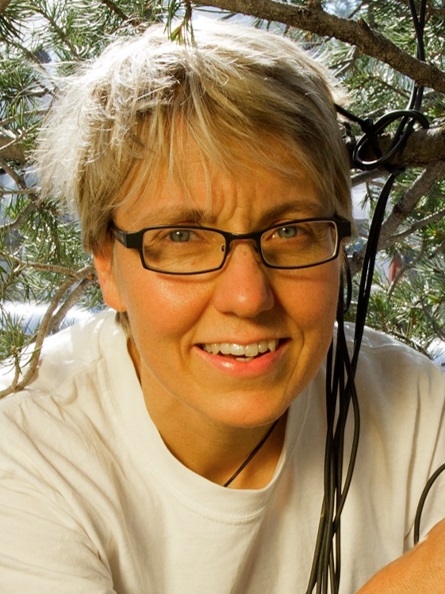
Sanna Sevanto
Sanna Sevanto has been named to the editorial board of Scientific Reports, an open-access, peer-reviewed Nature series journal and currently the fifth most-cited journal in the world. Drawn from experts in many different fields, Scientific Reports’ editorial boards are organized into categories ranging from earth sciences to biological and chemical sciences; Sevanto is a member of the board for environmental sciences. According to Scientific Reports, the editorial board “manages the peer review process and makes final decisions on whether papers should be accepted.”
Sevanto is an environmental physicist and team leader of Landscape and Ecosystem Dynamics and Resilience team in the Climate, Ecosystems, and Environmental Science group (EES-14) at Los Alamos National Laboratory. Her research focuses on vegetation-climate interactions and vegetation stress responses, with recent projects aiming at developing methods to produce microbial consortia to improve soil health and alleviate drought stress in plants. With over 90 influential peer-reviewed publications, she was ranked in the top 1% of most-cited scientists in her field by the Web of Science Database.
After receiving her doctoral degree in environmental physics from the University of Helsinki, Finland, Sevanto joined the organismic and evolutionary biology department as a postdoctoral fellow and visiting scientist at Harvard University. She then held a university lecturer position in meteorology at the University of Helsinki teaching atmospheric thermodynamics, fluid mechanics and environmental physics. She joined the Laboratory as a postdoctoral fellow in 2009 and became a research scientist at the Laboratory in 2012.
Capability Enhancement
CINT facility receives platinum-level sustainability certification
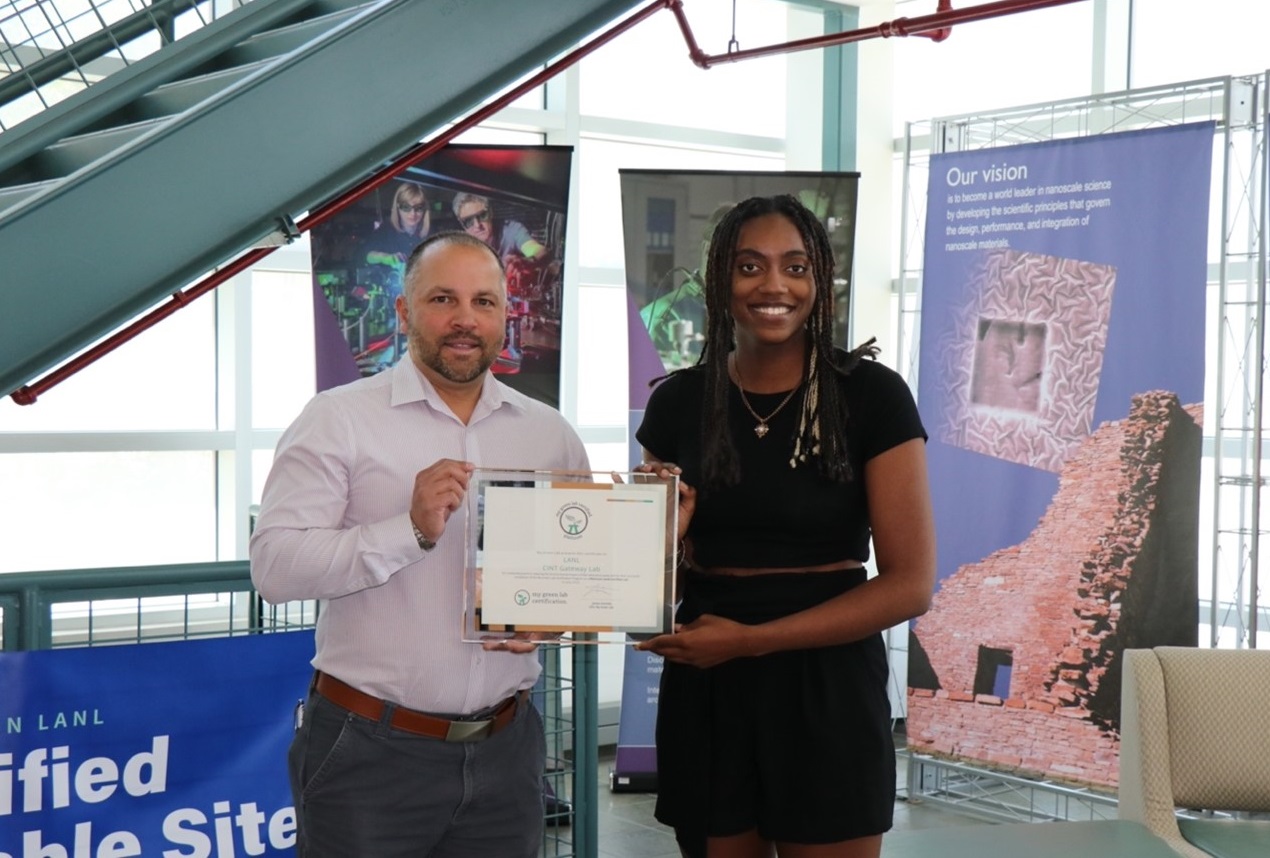
Ricardo Martí-Arbona (left) and CINT student Camari Knox (MPA-CINT) with CINT’s “My Green Lab” platinum certification. The certificate reads “to LANL CINT Gateway Facility for outstanding work in reducing the environmental impact of their laboratory space and for their successful completion of the My Green Lab Program as a Platinum Level Certified Lab in July 2023.” Knox was essential in helping CINT complete Phases 1 and 2 of the certification process.
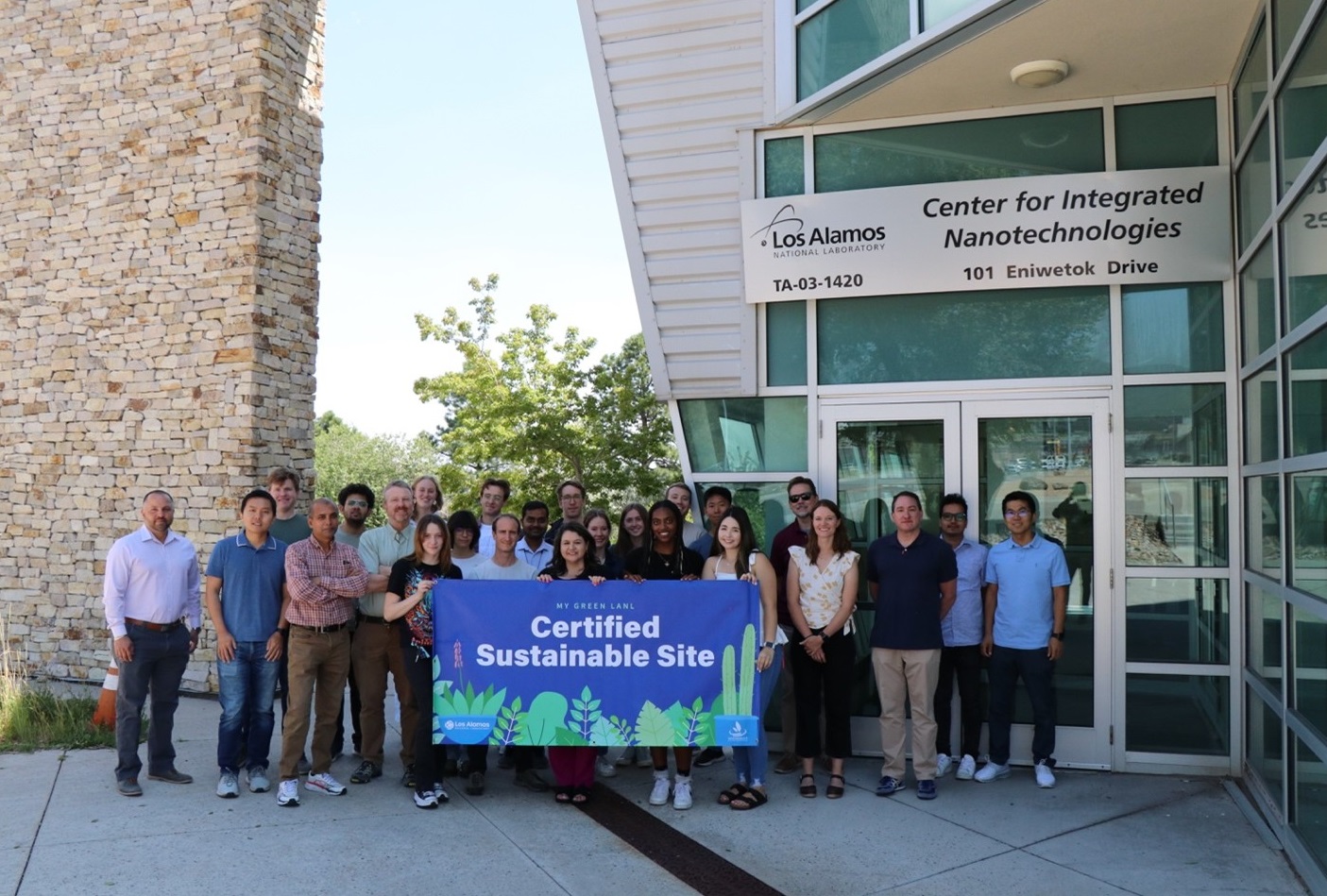
Members of the Center for Integrated Nanotechnologies with the banner proclaiming the center’s “My Green LANL” certification, a key step in the recent achievement of becoming My Green Lab platinum-level certified.
The Center for Integrated Nanotechnologies (CINT) Gateway facility at Los Alamos recently earned “My Green Lab” platinum-level certification. This endorsement is the second highest category achievable in the world-wide program recognized by the United Nations Race to Zero climate campaign as a key measure of progress toward a zero-carbon future. Considered the gold standard for lab sustainability best practices, the nonprofit certification program involves scientists in minimizing their environmental impacts and identifies waste-reduction opportunities so more is invested in science.
The CINT Gateway, a combination research and office building complex, features 11,000 square feet of lab space for materials synthesis, characterization, fabrication and advanced computation. CINT is a DOE Office of Science Nanoscale Science Research Center. Its researchers focus on providing the scientific basis for integrating nanoscale materials and enhancing materials performance.
CINT’s platinum-level efforts expands on sustainability practices underway when the facility earned My Green LANL certification in 2022. The program’s 2023 assessment noted that, in particular, CINT stood out for sustainable practices across a number of areas:
- Large equipment: The facility is dedicated to using shared printers; checking and maintaining high pressure lines or vacuum lines for leaks; turning off vacuum pumps when not in use; and powering down rooms or environmental spaces not in use.
- Waste management: The CINT Gateway takes advantage of the Lab’s recycling programs, and, when feasible, reuses disposable plastic and glass and minimizes single-use items.
- Resource management: The facility maintains an inventory of reagents, chemicals, laboratory supplies and equipment and shares equipment with colleagues rather than purchasing duplicates.
- Community: The facility communicates and engages with others about CINT’s sustainability goals and efforts; trains staff using common equipment or space on relevant sustainability best practices; and trains new hires and visiting researchers on lab sustainability best practices.
Mission
The work supports the Energy Security mission area and the Materials for the Future capability pillar.
Technical contact: Ricardo Martí-Arbona (C-DO)
Earth and Environmental Sciences
Full-wave inversion study named best paper by journal
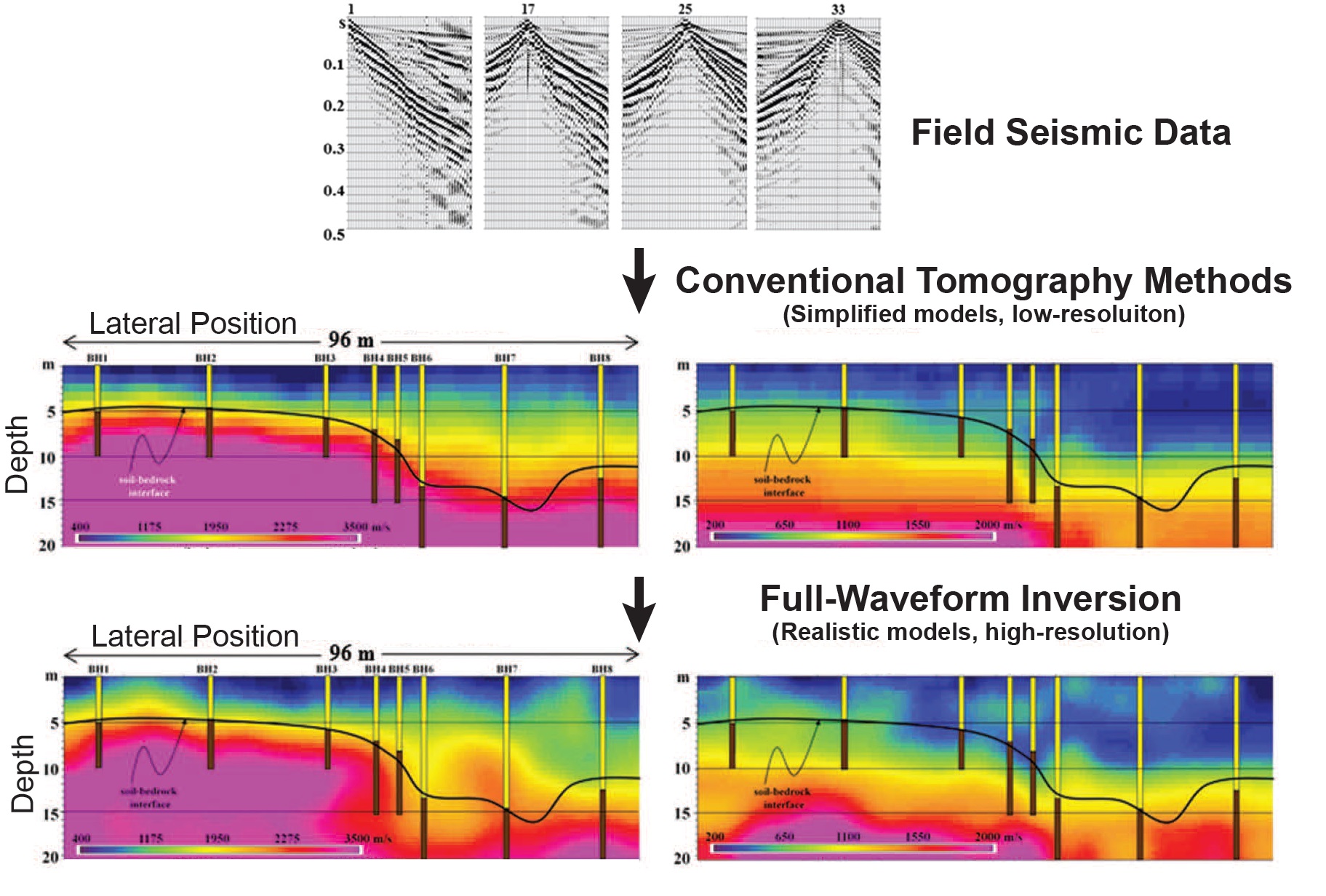
Full-waveform inversion exploits the full-wavefield information in field seismic data to derive high-resolution subsurface parameter models with realistic complexity that cannot be well recovered by conventional tomographic methods based on simplified physics models.
The Society of Exploration Geophysicists named a paper co-authored by Los Alamos National Laboratory scientists Lianjie Huang and Kai Gao (both EES-16) the best paper of 2022 in its journal, The Leading Edge. The paper, “A reality check on full-wave inversion applied to land seismic data for near-surface modeling,” used a field experiment to evaluate the performance of traveltime tomography and the technique known as full-wave inversion for near-surface seismic modeling, arriving at important recommendations for applying full-wave inversion to land seismic data for near-surface modeling.
While traveltime tomography possesses an advantage of computational efficiency compared to full-wave inversion, the latter technique is thought to be a viable alternative in cases where traveltime tomography fails to resolve velocity reversal. Full-wave inversion may also resolve certain lateral velocity variations. In the field experiment, the team used eight boreholes drilled to a 20-meter depth, recording 49 shot gathers along the line traverse, each with 48 traces. The team used a common-receiver-spread recording geometry and vertical-component geophones to acquire data.
A P-wave velocity-depth model for the near-surface and an S-wave velocity-depth model for the near-surface were estimated via traveltime tomography and Rayleigh-wave inversion, respectively. They were the initial models for the full-wave elastic inversion. The results of the full-wave elastic inversion showed an influence on the S-wave velocity estimation from the Rayleigh waves. The P-wave velocity estimation was influenced by the weak-amplitude P-waves. The authors conclude their paper with a number of recommendations for those interested in applying full-wave inversion to near-surface seismic modeling.
The Leading Edge’s best paper nominations are solicited from the Society of Exploration Geophysicists’ membership at large, voted on by the respective editorial boards and presented to the society’s board for final approval.
Funding and mission
The work supports the Global Security mission area and the Complex Natural and Engineered Systems capability pillar.
Reference
“A reality check on full-wave inversion applied to land seismic data for near-surface modeling,” The Leading Edge, 41, 1 (2022); DOI: 10.1190/tle41010040.1. Authors: Öz Yilmaz (Anatolian Geophysical), Kai Gao and Lianjie Huang (Los Alamos National Laboratory), Milos Delic (3GeoTomo), Jianghai Xia (Zhejiang University of China), Hossein Jodeiri (Laurentian University), and Andre Pugin (Geological Survey of Canada).
Technical contact: Kai Gao and Lianjie Huang (EES-16)
Experimental Capability Enhancement
Experimental platform leveraged for investigations at National Ignition Facility
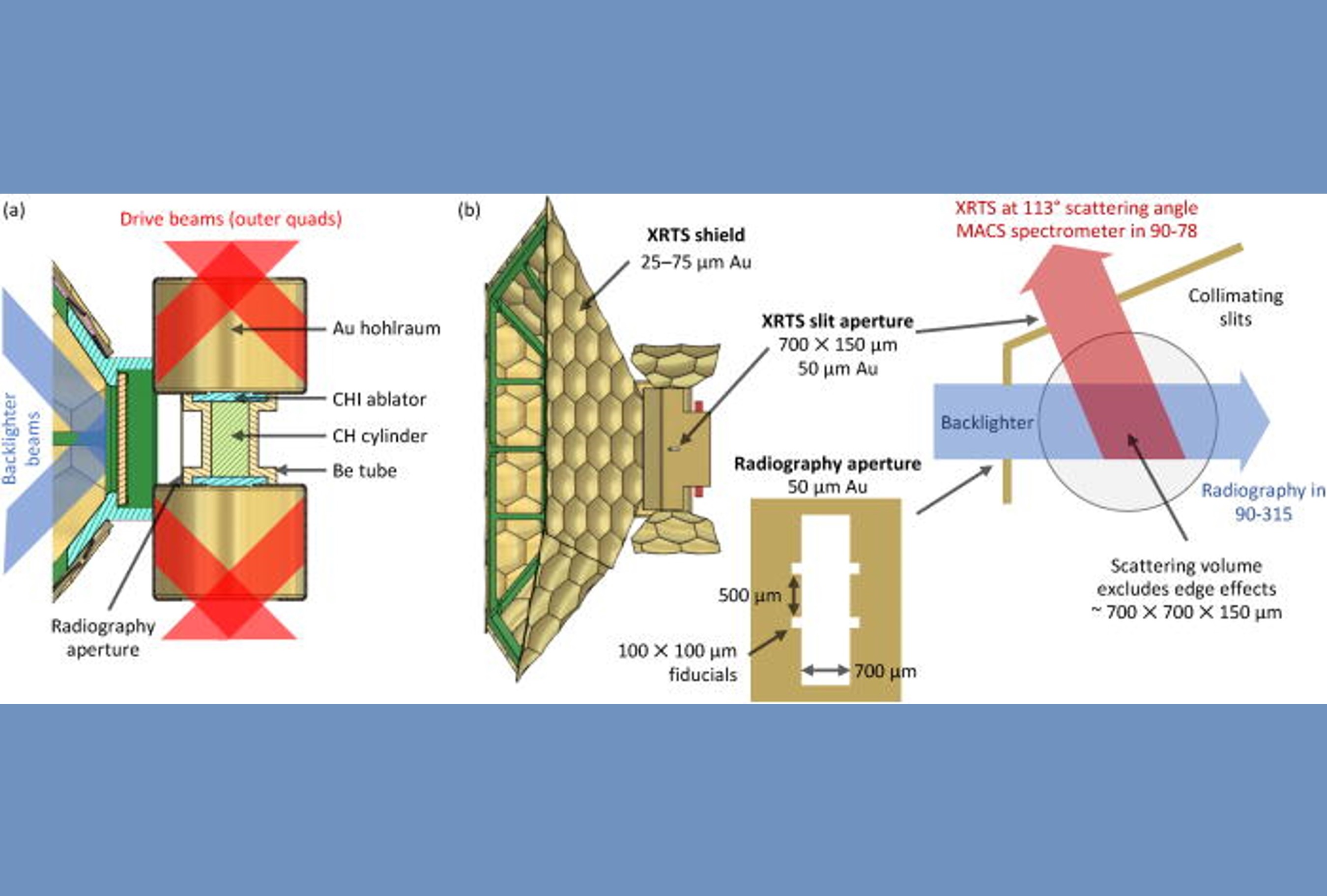
Caption: (a) Shown in a schematic, the internal target components of the collider planar shocks platform that allow for the capabilities of simultaneous XRTS and radiography measurements. (b) Shows the entire target, including radiography and XRTS apertures.
An experimental platform at the National Ignition Facility (NIF) uses colliding planar shocks to create warm dense matter with uniform conditions and enables high-precision equation of state measurements. The development of the platform furthers efforts to leverage programmatic work at the NIF, allowing universities and other researchers to access a large national resource to do cutting-edge science. The colliding planar shocks platform was described in Physics of Plasmas, work selected as an Editor’s Pick by that journal.
Accurate equation of state models are essential to understanding a material’s properties and for performing high-fidelity simulations. In the warm dense matter regime, equation of state models — models that account for the relationship between density, temperature and ionization in the regime — are required to predict the interior structure and evolution of astrophysical bodies and are critical in the design of inertial confinement fusion implosions. Yet such data is difficult to model in this regime due to its complexity. While high-quality experimental benchmarks are essential to guide models, little experimental data exists.
The result of a years-long effort, the colliding planar shock experimental platform was designed using a combination of several previously successful platforms at the NIF. The design of the X-ray Thomson scattering shielding was developed for X-ray scattering measurements on the National Ignition Facility’s gigabar platform. The physics package, laser drive and radiography setup use the fundamental design of the shock/shear platform developed at Los Alamos National Laboratory, with the core physics package and drive platform developed nearly a decade ago to study hydrodynamic instabilities in the high energy density regime relevant to inertial confinement fusion physics.
The colliding planar shocks/equations of state experiment is the latest to use a derivative of the shock/shear platform. In the experiment, the nominal beryllium tube was shortened and filled with a solid polystyrene cylinder to create quasi-steady-state and uniform condition of interest in the shocked solid polystyrene long enough to make the measurement. Los Alamos brings a unique capability to the project as the only Department of Energy laboratory that can supply machined beryllium parts. Beryllium is the only material strong enough to serve as a shock tube, yet transparent enough to allow scattered X-rays to be collected via Thomson scattering.
Comparing hydrodynamic simulations to X-ray radiography data from initial experiments studying hydrocarbons, the researchers demonstrated plasmas with uniform densities within plus or minus 25% of the average probed condition.
The work leverages Los Alamos expertise in high energy density science, inertial confinement fusion, target fabrication, and large-scale modeling of the platform using Laboratory supercomputers.
Funding and mission
The Los Alamos portion of the work was funded by the U.S. Department of Energy National Nuclear Security Administration’s Office of Experimental Science and the NIF Discovery Science Program. The research supports the Laboratory’s Global Security mission and its Nuclear and Particle Futures capability pillar.
Reference
“The colliding planar shocks platform to study warm dense matter at the National Ignition Facility,” Physics of Plasmas 30, 062701 (2023); DOI: 10.1063/5.0146624. Authors: M. J. MacDonald, T. Döppner, D. Kalantar, S. J. Ali, P. M. Celliers, R. Heredia, J. A. Gaffney, A. M. Saunders and R. Zacharias (Lawrence Livermore National Laboratory); C. A. Di Stefano, K. A. Flippo, E. C. Merritt, D. W. Schmidt and C. T. Wilson (Los Alamos National Laboratory); L. B. Fletcher and S. H. Glenzer (SLAC National Accelerator Laboratory); S. Vonhof (General Atomics); G. W. Collins (University of Rochester); D. O. Gericke (University of Warwick); D. Kraus (University of Rostock); R. W. Falcone (University of California, Berkeley).
Technical contact: Kirk Flippo (P-2)
Materials Physics and Applications
‘Goldilocks’ approach brings ‘just right’ water management to unitized reversible fuel cells
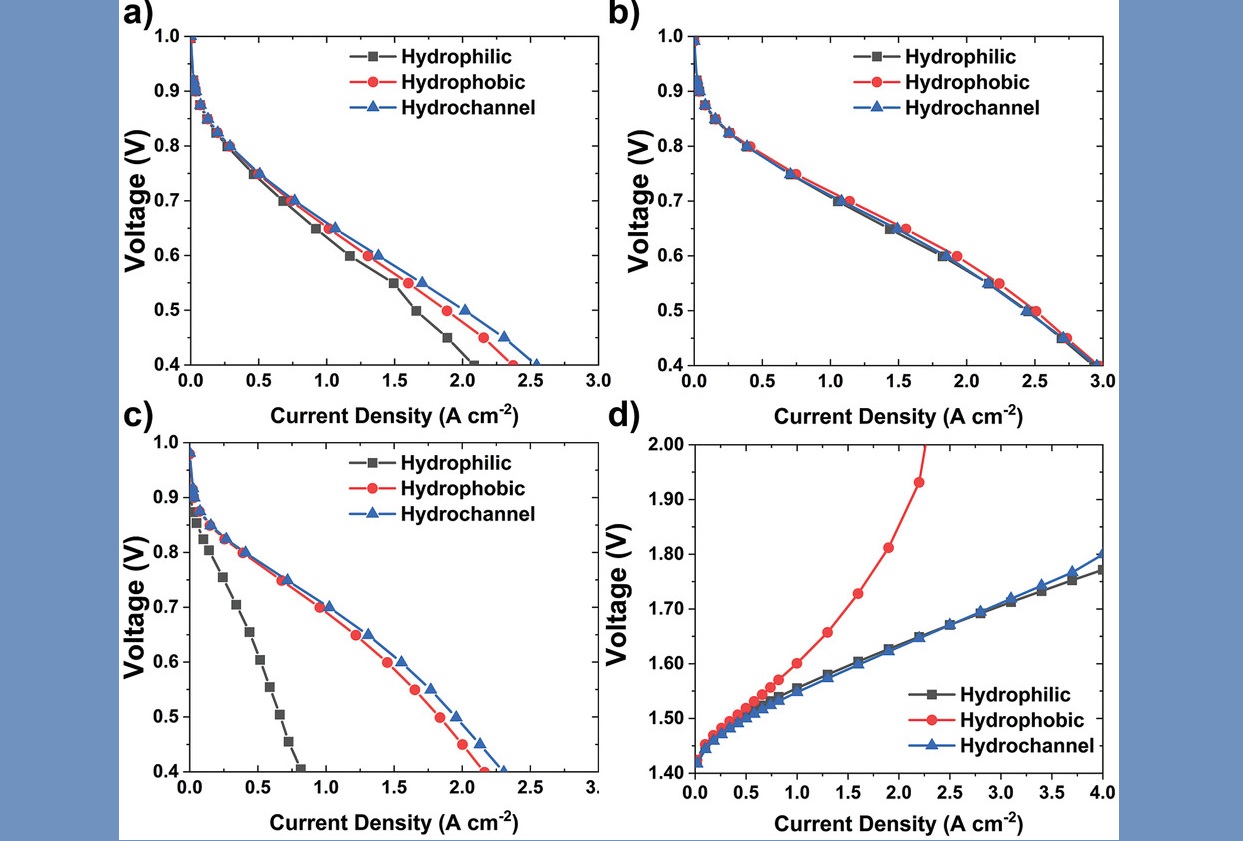
Polarization curves in fuel cell mode at a) 75% RH, b) 100% RH, c) 150% RH, and d) water electrolyzer mode for cells with hydrophilic, hydrophobic, and hydrochannel porous transport layers. As the figure shows, the hydrochannel PTL approach provides an optimal combination of high performance in both fuel cell and electrolyzer mode. Image published in Advanced Energy Materials, used via Creative Commons license.
Much like the Goldilocks of folklore, Los Alamos National Laboratory researchers in collaboration with external colleagues have struck upon a “just right” method for improved water management in unitized reversible fuel cells (URFCs), resulting in record-high round-trip efficiency.
Combining the functionality of a fuel cell and a water electrolyzer in one electrochemical device, URFCs could play a crucial role in the large-scale storage of intermittent, renewable energy from sources such as from wind and solar, provided the conflicting technological needs of their operation can be overcome. To function optimally, URFCs require opposing liquid water saturation requirements — one high when in electrolyzer mode and one low in fuel cell mode.
In research published in Advanced Energy Materials, the team reports a new approach to URFC design based on hydrochannel porous transport layers (PTLs). These layers provide hydrophilic channels for water transport through an otherwise hydrophobic PTL. Hydrochannel PTLs enable effective water management in both URFC operation modes, demonstrating unprecedented performance and round-trip efficiency, as confirmed by neutron radiography measurements of water content in both operating modes. This research draws on Los Alamos’ unique capabilities in microfabrication for electrode and transport media development, which is part of the longstanding hydrogen and fuel cell program at the lab.
The researchers noted that while these hydrochannel PTLs provide higher performance and round-trip efficiency compared to conventional PTLs, further improvements in hydrochannel PTL design could enable still greater performance enhancements. In future work, the researchers plan to investigate the roles of PTL fiber diameter, porosity, and pore size distribution in controlling water and gas transport in hydrochannel PTLs.
Funding and mission
This work was funded by the DOE Office of Energy Efficiency and Renewable Energy, Hydrogen and Fuel Cell Technologies Office and by the Laboratory Directed Research and Development program at Los Alamos. The research supports the Energy Security mission area and the Materials for the Future capability pillar.
Reference
“A Goldilocks approach to water management: Hydrochannel porous transport layers for unitized reversible fuel cells,” Advanced Energy Materials, 13, 161, 2203952 (2023); DOI: 10.1002/aenm.202203952. Authors: S. Komini Babu, A. Yilmaz, M. A. Uddin and J. S. Spendelow (Los Alamos National Laboratory); A. Yilmaz and U. Pasaogullari (University of Connecticut); and J. LaManna, E. Baltic and D. L. Jacobson (National Institute of Standards and Technology).
Technical contact: Jacob Spendelow (MPA-11)
Materials Science and Technology
Quantifying additive manufacturing could help repair high-value components
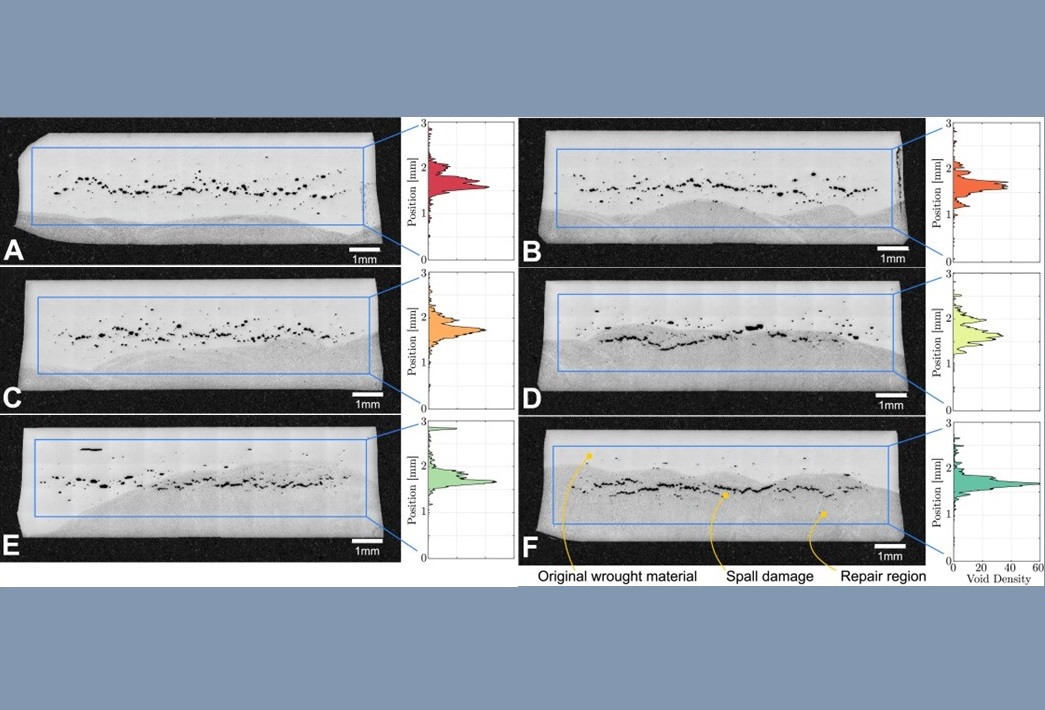
Optical microscopy images show the impact face (top surface) in each sample. Original wrought material appears lighter in color while the repair region is darker and shows a fish-scale-like pattern. The spall plane is evident in the center of each sample. Charts to the right indicate the net damage in the vertical direction across the region (indicated by the blue rectangular box in the microscopy image).
Additive manufacturing (AM) has the potential to be used for repair of high-value parts, and thus avoiding challenges associated with low production volume and high startup costs. However, the strength and integrity of such repaired parts, especially under dynamic loading, is still largely unknown. To employ AM technology in repair applications requires quantifying the process-structure-properties-performance relationship — that is, understanding how these components interconnect to affect the material’s ultimate behavior.
In Acta Materialia, Los Alamos materials researchers present the results of additively repaired stainless steel samples subjected to high strain-rate dynamic tests — addressing key elements not covered in existing published research on AM repairs. Despite minor differences in the materials’ properties, the overall dynamic response of the original material and repaired samples was similar, suggesting that AM is a promising approach for repairing high-value components.
In the study, the researchers created intentional surface defects of varying sizes in stainless steel samples. The surface damage was repaired using electron beam additive manufacturing. The repaired samples were then subjected to high strain-rate impacts using a gas-gun launched flyer plate, with sample velocity measured using photon Doppler velocimetry. The recovered samples then underwent optical microscopy and electron backscatter diffraction analysis. This analysis clearly showed that overall the equation of state and failure in the repaired components was similar to the un-paired components. However, as the defect depth was increased and this repaired region coincided with the region of maximum tension, changes in the void morphology were observed. This suggests that there might be a critical volume fraction of a component that that can be repaired before the dynamic properties are altered.
Future research efforts may focus on alternative processing parameters or post-repair treatments to achieve a more homogeneous grain structure and better match the behavior of the repair to the original material.
The work leverages Sigma Division’s manufacturing science expertise and capabilities — particularly its machining and additive repair operations — and the Center for Integrated Nanotechnologies’ quasi-static and dynamic materials diagnostic and dynamic behavior of materials experimental capabilities and expertise.
Funding and mission
This work, funded by U.S. Department of Energy National Nuclear Security Administration, supports the Materials for the Future capability pillar.
Reference
“Dynamic properties of 316L stainless steel repaired using electron beam additive manufacturing,” Acta Materialia 246, 118636 (2023); DOI: 10.1016/j.actamat.2022.118636. Authors: Jesse G. Callanan, Amber N. Black, Samantha K. Lawrence, David R. Jones, Daniel T. Martinez, Ramon M. Martinez and Saryu J. Fensin (Los Alamos National Laboratory).
Technical contact: Saryu Fensin (MPA-CINT)
Physics
First-ever X-ray scattering experiments on plutonium allotropes at NSLS-II
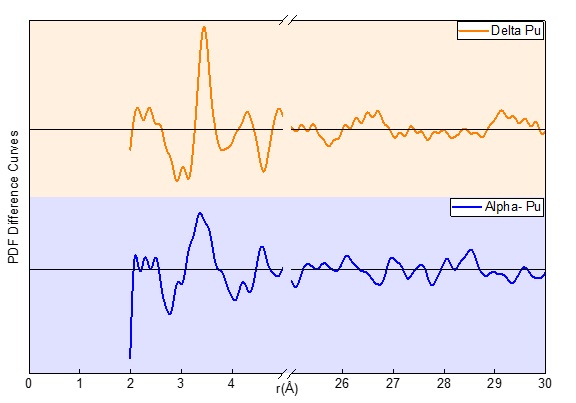
The pair distribution function difference curves correspond to the subtraction of the experimental data and model fits to said experimental data. For both delta and alpha plutonium, the most local regions (approximately 2-5 angstrom) oscillate around the zero-line (black line) with greater amplitude than the more long-range regions (approximately 25-30 angstrom). This is strongly indicative of disparate short- and long-range crystal structures for the respective allotropes.
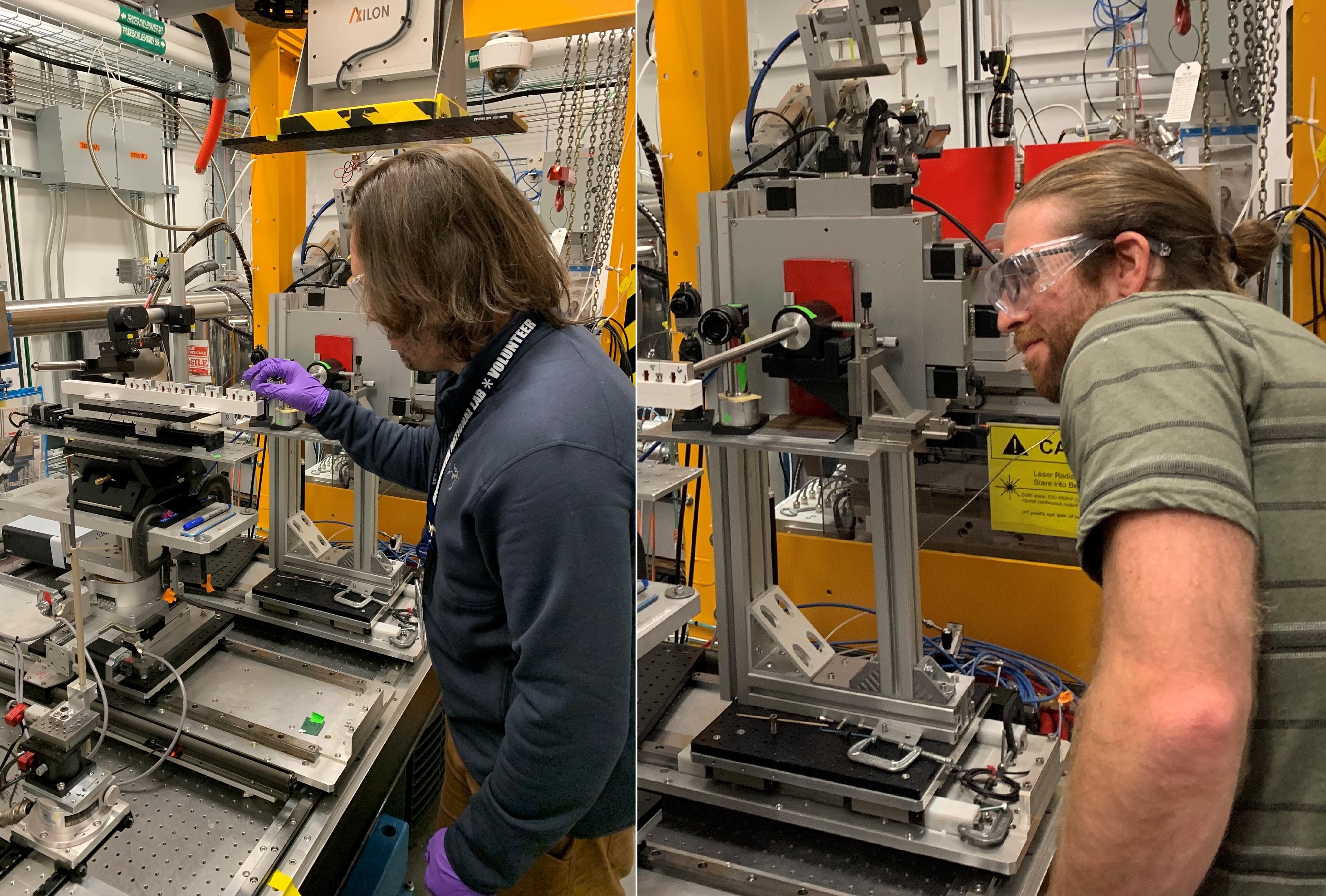
Phelan (left) and Cook (right) loading and inspecting sample assemblies, respectively, on a modified goniometer stage designed by Arellano (not pictured) within a hutch on the 28-ID-1 beamline.
A team from Los Alamos National Laboratory including W. Adam Phelan (scientist, MST-16), David C. Arellano (research technologist, MST-16), and Matthew S. Cook (postdoctoral research associate, MPA-Q transitioning to MST-16) recently conducted experiments at the National Synchrotron Light Source-II’s 28-ID-1 beamline, located on the Brookhaven National Laboratory campus. While external user experiments commonly take place at beamline 28-ID-1 and at other beamlines comprising the NSLS-II synchrotron facility, these experiments were first-of-a-kind for this specific light source. At NSLS-II, Phelan and colleagues became the first user group to perform X-ray scattering experiments on plutonium allotropes.
The overall objective for these studies was to glean insight into the differing U.S. and Russian plutonium-gallium phase diagram. At 28-ID-1 the research team searched for subtle features in differently heat- and pressure-treated plutonium samples via an X-ray scattering method known as X-ray pair distribution function. Simply stated, the pair distribution function technique is commonly used to explore a material’s “local-” or “short-range” crystal structure, while the corresponding diffraction method probes the “average” or “long-range” crystal structure.
The team’s studies will inform an understanding of the aging of plutonium alloys important to Los Alamos mission spaces. While the post-analysis is preliminary, both alpha- and delta-plutonium have obvious differences in their respective short- and long-range crystal structures. This is highlighted in the figure below. Encouraged by the initial results, the team is making plans for a return visit to perform additional experiments.
Funding and mission
The work was supported by the Laboratory Directed Research and Development program at Los Alamos National Laboratory. The work supports the Global Security mission area and the Materials for the Future capability pillar.
Technical contact: W. Adam Phelan (MST-16)