Science Highlights, November 9, 2016
Awards and Recognition
Capability Enhancement
Prototype Red Sage experiments improve design and diagnostics
Novel liquid helium technique aids search for a neutron electrical dipole moment
Earth and Environmental Sciences
Accounting and risk analysis for CO2 sequestration at enhanced oil recovery sites
Materials Physics and Applications
Engineering nanoscale metamaterials using a novel self-assembly approach
Materials Science and Technology
First-ever rare-earth free permanent magnets with 20 MGOe energy product
Awards and Recognition
Laboratory inventions receive R&D 100 Awards
A panel of judges selected by R&D Magazine has chosen five Los Alamos innovations for 2016 R&D 100 Awards and an additional one for Special Recognition for Green Technology. These prestigious “Oscars of Invention” honor the top technological advances of the past year. R&D 100 Awards span industry, academia, and government-sponsored research. The Awards demonstrate the continued success of Laboratory researchers in technical innovation for national security science. This year’s honorees are the result of partnerships with private industry, universities, and other national laboratories, and illustrate many possible paths from scientific creativity to deployment of solutions. These developments also benefit the nations’ industrial competiveness. Entropy Engine and PathScan are now commercially available products for cybersecurity applications, Carbon Capture Simulation Initiative (CCSI) Toolset supports carbon dioxide capture technology, and industry benefits from Virtual Environment for Reactor Applications (VERA) to model the performance of light water reactors.
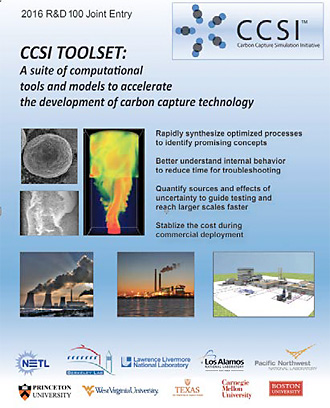
CCSI (Carbon Capture Simulation Initiative) Toolset is a suite of computational tools and models that supports and accelerates the development, scale-up, and commercialization of carbon dioxide (CO2) capture technology to reduce domestic and global carbon dioxide emissions. The invention addresses key industrial challenges, including developing a baseline for the uncertainty in simulation results, quantifying and reducing the level of uncertainty in processes, and options, enhances understanding of how processes will operate at scale under relevant field conditions, and increases understanding of how uncertainty affects risk. It is the only suite of computational tools and models specifically tailored to help maximize learning during the scale-up process in order to reduce risk. Maximizing the learning during pilot projects reduces the time needed for subsequent commercialization and enables greater investment confidence. The CCSI Toolset could also be employed in related industries to enable faster, more cost effective scale up of other new technologies. The National Energy Technology Laboratory submitted the joint entry with Lawrence Berkeley National Laboratory, Lawrence Livermore National Laboratory, LANL, Pacific Northwest National Laboratory, Princeton University, West Virginia University, University of Texas at Austin, Carnegie Mellon University, and Boston University. Joel Kress (Physics and Chemistry of Materials, T-1) led the Los Alamos team, which included Jim Gattiker, Sham Bhat, and Peter Marcy (Statistical Sciences, CCS-6); Brett Okhuysen (Systems Design and Analysis, NEN-5); David DeCroix (Intelligence and Emerging Threats Program Office, GS-IET), and Susan Sprake (Richard P. Feynman Center for Innovation, FCI-DO). Technical contact: Joel Kress
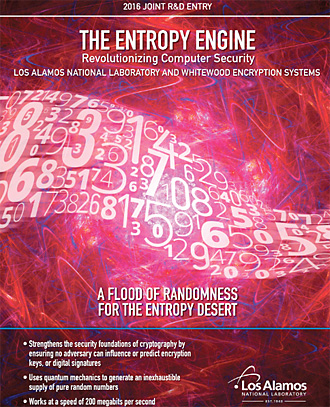
Entropy Engine is a random number generator that addresses a key fundamental flaw in modern crypto systems: predictability. The invention strengthens the foundation of computer security by producing an inexhaustible supply of pure random numbers at speeds of 200 million bits per second. Entropy Engine uses the unique properties of quantum mechanics to generate true entropy (random numbers) in a way that makes it immune from all external influences. The behavior of the universe at the smallest scale – the quantum level – is fundamentally unpredictable and makes the immunity possible. The new technology supplies a flood of trustworthy, verifiable truly random numbers, making it virtually impossible for even the most sophisticated cyber attackers to break into the assets protected by this technology.
Los Alamos submitted Entropy Engine as a joint entry with Whitewood Encryption Systems. Raymond Newell (Applied Modern Physics, P-21) led the Los Alamos team of Glen Peterson (P-21) and David Guenther (Space Electronics and Signal Processing, ISR-4), with collaborators Richard Moulds (Whitewood Encryption Systems), Jane E. Nordholt and Richard Hughes (retired Laboratory employees); Robert Van Rooyen (Summit Scientific Inc.), and Alex Rosiewicz (A2E Partners, Inc.). Technical contact: Raymond Newell
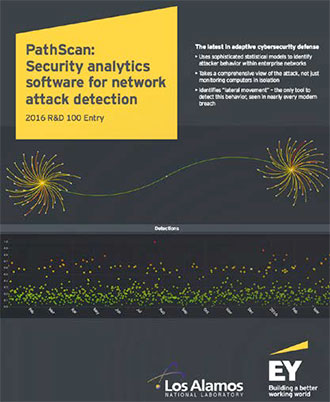
PathScan provides security analytics for computer network attack detection. Traditional computer network security tools, which search for malware or network signatures, insufficiently protect from expensive data breaches. Traditional defense mechanisms of perimeter controls and end-point antivirus protection cannot keep pace with these increasingly innovative and sophisticated adversaries. Rather than detecting something that “looks” like a cyberthreat, PathScan searches for anomalous communications behavior within the network. The invention performs a statistical analysis of abnormal behavior across a network and identifies the lateral, reconnaissance, and data staging behaviors of attackers. This situational awareness enables network defenders to identify hosts in the network that have been co-opted by the adversary and hone in on potential attack behavior before it can wreak havoc.
Ernst & Young submitted PathScan, a joint entry with LANL, based on technology licensed from the Lab. Michael Fisk (Office of the Chief Information Officer, OCIO) led the Los Alamos team of Curtis Storlie (Statistical Sciences, CCS-6), Alexander D. Kent (Intelligence and Emerging Threats Program Office, GS-IET), and Melissa Turcotte (Advanced Research in Cyber Systems, A-ARCS). Ernst & Young inventors include Joshua Neil, Curt Hash, Ben Uphoff, Alexander Brugh, Matt Morgan, and Joseph Sexton. Technical contact: Michael Fisk
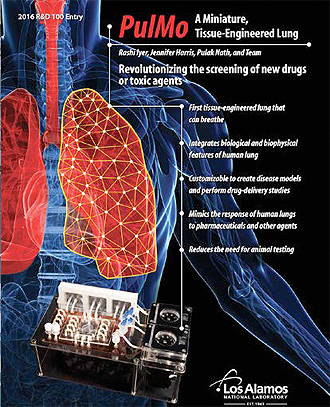
PuLMo (Pulmonary Lung Model) is a miniature, tissue-engineered lung developed to revolutionize the screening of new drugs or toxic agents. Current screening methods often use different types of animals, which may not be accurate predictors of the response in humans. PuLMo is 1/1000th the size of an actual human lung. Such miniaturization makes it possible to evaluate multiple units at a time and to create models for different lung specific disease conditions. PuLMo could be used as a platform to study the flow dynamics of particles inside a lung for applications in drug delivery and particle/pathogen deposition studies. It could also serve as a realistic multicellular pulmonary model for performing fundamental research to understand disease morphology and host-pathogen interactions. The invention has the potential to make screening of new drugs more effective by improving the reliability of pre-clinical testing and saving time, money, and lives. PuLMo could reduce and eventually eliminate the use of animals in pharmaceutical testing.
Rashi S. Iyer (Information Systems and Modeling, A-1) led the team of Pulak Nath (Applied Modern Physics, P-21), Jennifer Foster Harris, Ayesha Arefin, Yulin Shou, Kirill A. Balatsky, and Jen-Huang Huang (Biosecurity and Public Health, B-10); Srinivas Iyer (Bioscience Division Office, B-DO); Jan Henrik Sandin (Instrumentation and Controls, AOT-IC); David Platts and John Avery William Neal (P-21); Timothy Charles Sanchez (Bioenergy and Biome Sciences, B- 11); and Miranda Huang Intrator (Richard Feynman Center for Innovation, FCI-DO). Technical contact: Rashi Iyer
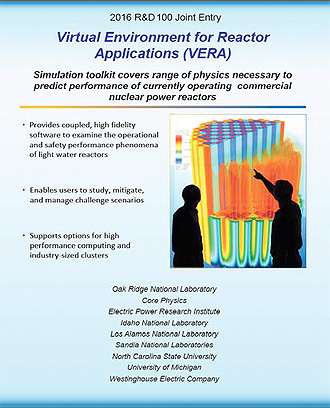
Virtual Environment for Reactor Applications (VERA) provides coupled, high fidelity, software capabilities to examine light water reactors’ operational and safety performance-defining phenomena at levels of detail previously unattainable. The multiphysics simulation toolkit covers the range of physics necessary to predict the performance of currently operating commercial nuclear power reactors. This capability enables users to study, mitigate, and manage the challenge problems identified by the industry to a level of understanding that is not available through other toolsets. VERA supports options for both high performance computing and industry-sized computing clusters in a manner that is accessible and easily understood for most users.
Oak Ridge National Laboratory submitted VERA, a joint entry with Core Physics, Electric Power Research Institute, Idaho National Laboratory, Los Alamos National Laboratory, Sandia National Laboratories, North Carolina State University, University of Michigan, and Westinghouse Electric Company. Christopher Stanek (Materials Science in Radiation and Dynamics Extremes, MST-8) led the Los Alamos work. Technical contact: Christopher Stanek
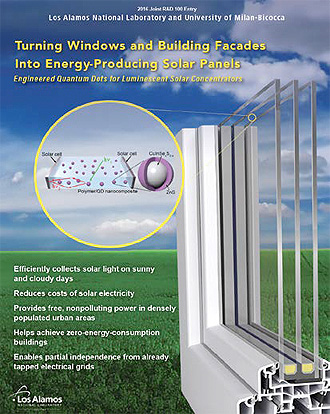
Turning Windows and Building Facades into Energy- Producing Solar Panels: Engineered Quantum Dots for Luminescent Solar Concentrators won a Special Recognition Award for Green Technology.
These revolutionary semitransparent windows contain highly emissive semiconductor nanocrystals (quantum dots) that collect sunlight for edge-installed photovoltaics and provide a desired degree of shading. The material can turn windows and building facades into electrical generators of nonpolluting power. The dots absorb the sunlight, re-emit it at a longer wavelength, and waveguide it towards edge installed photovoltaic cells for production of electricity. As a result of this technology, once passive building facades could be transformed into power-generation units, which would be particularly useful in densely populated areas. Unlike other solar energy harvesting technologies with quantum dots, a key element of this technology involves the use of nontoxic copper indium selenide sulfide (CuInSexS2-x) quantum dots. This material is easily fabricated and processed into devices that enable the highly efficient harvesting of broadband solar radiation, provide a perfect spectral match to silicon photovoltaics, and prevent alteration of color perception.
Los Alamos submitted the joint entry with the University of Milano-Bicocca as a co-developer. Victor I. Klimov (Physical Chemistry and Applied Spectroscopy, C-PCS) led the team of Kirill Velizhanin (Physics and Chemistry of Materials, T-1), Hunter McDaniel (former LANL Postdoctoral Researcher, currently with UbiQD LLC), Sergio Brovelli and Francesco Meinardi (University of Milano-Bicocca). Technical contact: Victor Klimov
Since 1978 when it first competed, Los Alamos has won 137 of the prestigious Awards. Information about the Lab’s past R&D 100 Awards here. Technical contact: Janet Mercer-Smith
Iain May honored with Outstanding Achievement Award
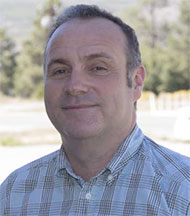
The NNSA honored Iain May (Inorganic, Isotope and Actinide Chemistry, C-IIAC) for work towards ensuring the reliability and domestic supply of molybdenum-99 (Mo-99), a medical isotope used for diagnostic imaging. Peter Hanlon (Assistant Deputy Administrator, Office of Material Management and Minimization) cited May for “outstanding achievement in support of the Office of Material Management and Minimization’s Molybdenum-99 Program and in the minimization of highly enriched uranium in civilian applications. Your work with uranium detection technologies and project management support has been critical to the significant progress made in the development of low enriched uranium fission-based molybdenum-99 production technologies.”
Mo-99 is the parent isotope of technetium-99, which is used in nuclear medicine worldwide to formulate radiopharmaceuticals that diagnose heart disease, cancer, neurologic disease and other applications. The radioisotope is used in approximately two-thirds of all nuclear medicine imaging procedures, amounting to roughly 50,000 diagnostic nuclear medicine procedures performed every day in the United States. Until recently, the entire U.S. supply of Mo-99 for nuclear medicine has been produced in aging foreign reactors using proliferation-sensitive highly enriched uranium (HEU) targets. Maintenance and repair shutdowns of these reactors have significantly disrupted the supply of Mo-99 in the U.S. and much of the rest of the world.
Supply disruptions and ongoing nonproliferation and domestic supply concerns spurred the efforts to develop an alternative production capability of Mo-99 in North America. NNSA has been funding work to minimize and eliminate the use of HEU to produce Mo-99 and develop a U.S.-based production capability that has been absent since 1989.
May’s award from NNSA recognized his development of a spectroscopic analysis technique to measure uranium concentration in unknown solutions. His research aims to accelerate the domestic production of Mo-99 using low enriched uranium rather than HEU. May accepted the award from Jeff Chamberlin, director of the NNSA Office of Conversion, and Rilla Hamilton, the NNSA Mo-99 program director, at the 2016 Mo-99 Topical meeting in St. Louis.
May received a PhD in inorganic chemistry from Durham University in the United Kingdom. He joined the Laboratory in 2007. His work supports the Lab’s Global Security mission area and the Science of Signatures and Materials for the Future science pillars. Technical contact: Iain May
Susan Coulter elected chair of the OpenFabrics Alliance (OFA)
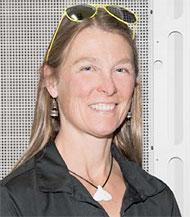
The OpenFabrics Alliance (OFA) has elected Susan Coulter (High Performance Computing Design group, HPC-DES) as chair. The open source-based organization develops, tests, licenses, supports, and distributes OpenFabrics networking software. This software is widely used with high performance computing and enterprise data center applications. In her new role as chair, Coulter will continue building collaborations between members of the Alliance and helping define and implement the future of high speed networking.
Coulter received a B.S. in Computer Science from Missouri University of Science and Technology. She joined LANL in 1992. Her work in HPC Division has spanned many large system installations, including Roadrunner, the first HPC system to reach a speed of 1 PetaFLOP/s, Cielo, and Trinity, which is being installed today. Coulter has also played an integral role in the deployment of many smaller HPC systems, developed a variety of network management tools, and led HPC Division’s production networking team. Currently, she leads HPC network integration efforts in HPC-DES and is the Lab’s subject matter expert for InfiniBand. Her work supports the Lab’s Nuclear Deterrence mission area and the Information, Science, and Technology for Prediction science pillar. Technical contact: Susan Coulter
Bioscience
Microbes developed to degrade toxic organophosphates and glow
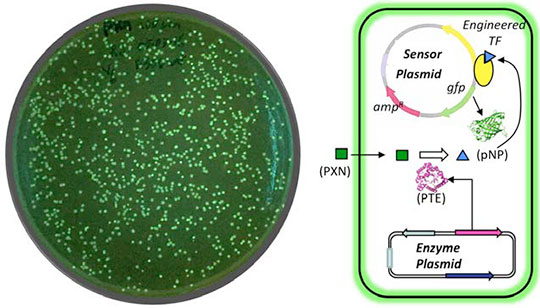
Figure 1. (Left): Glowing microbial colonies on growth media supplemented with the toxic organophosphate insecticide, paraoxon (PXN). (Right): Illustration of a microbial cell and sequence of events resulting in a fluorescent signal. PTE (phosphotriesterase) is an enzyme that degrades PXN. The degradation product of paraoxon is pNP (p-‐ nitrophenol), which activates an engineered transcription factor (TF) to express a green fluorescent protein (GFP). ampR is a ampicillin resistance gene that confers viability of the microbial cell in the presence of the antibiotic. Cells surviving on ampicillin confirm the presence of sensor plasmid, which also carries genes for TF and the reporter GFP.
Organophosphates are potent inhibitors of cholinesterase enzymes that affect neuromuscular function and can cause acute neurotoxic poisoning in humans and animals. This class of molecules includes insecticides and also dangerous nerve agents that could be used nefariously. To address threats posed by organophosphates, a team has engineered a microbe that can efficiently degrade these compounds and also emit light to indicate the presence of the toxic agents in the environment. The journal Nucleic Acids Research published the research.
The critical step in engineering a biosensor is the creation of a protein variant that can take a small molecule (ligand) as an input and generate a detectable signal (fluorescence) as an output. This is achieved by manipulating a specific family of regulatory proteins called transcription factors (TF). Organisms naturally evolve a wide variety of TFs to help them respond to threats by turning on production of certain genes. However, relatively new threats – such as human developed insecticides like organophosphates – have not been around long enough to evolve a way to recognize them.
Charlie Strauss (Bioenergy and Biome Sciences, B-11) led a LANL team that has developed a method to recognize the organophosphate insecticide paraoxon (PXN). First, the team engineered new variants of an existent native TF to respond to a product called p-nitrophenol (pNP), which is created when PXN is broken down. The engineered TF is sensitive to the presence of nonnatural ligand, pNP, almost 20-fold more than the sensitivity of native TF for the natural ligand, 4-hydroxybenzoate (4HB). The 4HB and pNP ligands share a single atom difference. The authors suggest that the difference in local negative charge distribution of the ligands could explain the specificity switch for the TFs. Other TF engineering efforts (for various molecules and using variety of scaffolds) only produced variants that were two to four orders of magnitude weaker in sensitivity than the pNP responsive one.
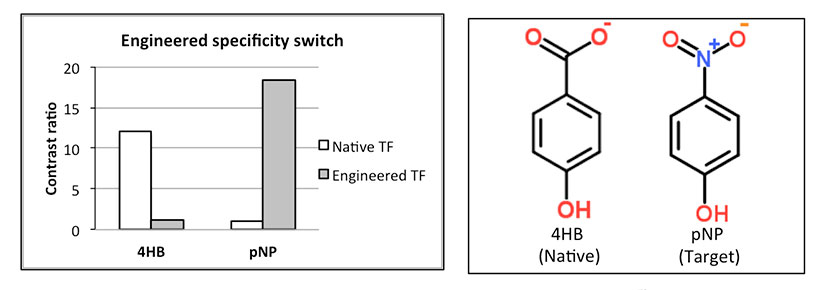
Figure 2. (Left): Ligand specificity switch observed between native and engineered TFs. Contrast ratio is the fold change in microbial fluorescence in the absence and presence (200 μM) of respective ligands. (Right): Molecular structure of 4HB and pNP show a single atom difference. Close to neutral pH, pNP is expected to be in equilibrium with a deprotonated hydroxyl group to give the p-‐nitrophenolate anion.
LANL’s TF engineering approach is a rare example in the protein-engineering world. A structure-based protein design approach substantially reduces the efforts to navigate through the astronomically-large sequence space. But the native TF structure was unknown and having structural information was imperative. Only very recently the homology modeling of proteins using a template with known structure has started to show atomic level accuracy, but no efforts had been made to engineer new proteins based on homology models. The LANL team aimed to create a homology model of the native TF, dock the native ligand in the putative binding pocket of the native TF, and understand the ligand-protein interaction before proceeding with the design and selection of a TF variant for the ligand of interest pNP. This approach found a pNP responsive TF. The team collaborated with Argonne National Laboratory to determine the ligand-bound x-ray structure of the native TF. The x-ray structure closely resembled the ligand-bound homology model. This result demonstrates a novel protein engineering approach, in which a structure-based protein design can be pursued in the absence of actual structural information of the protein.
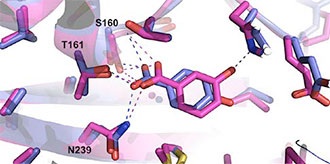
Figure 3. Comparison of the level of accuracy between a homology model and experimentally confirmed structure of the protein. Homology model of native TF with docked ligand 4HB (blue) aligned with an atomic level accuracy with the x-‐ray structure of the same protein bound to 3-‐hydroxybenzoate (magenta). The key interactions between ligand and protein observed in the homology model were conserved in the experimentally determined x-‐ ray structure. Dotted lines represent the polar interactions.
Reference: “A Microbial Sensor for Organophosphate Hydrolysis Exploiting an Engineered Specificity Switch in a Transcription Factor,” Nucleic Acids Research 44 (17), 8490 (2016); doi: 10.1093/nar/gkw687. Authors: Ramesh K. Jha, Theresa L. Kern, and Charlie E. M. Strauss (Bioenergy and Biome Sciences, B-11); Youngchang Kim, Christine Tesar, and Robert Jedrzejczak (Argonne National Laboratory); Andrzej Joachimiak (Argonne National Laboratory and University of Chicago).
The Defense Threat Reduction Agency funded the Los Alamos work on microbial sensor and organophosphate hydrolysis, the University of California Lab fee helped generate the idea and preliminary data on TF design for small molecule sensing, and the LANL Institutional Computing Program provided computing resources. The research supports LANL’s Global Security mission area and the Science of Signatures science pillar through the development of biosensors for toxic agents and the means to degrade these materials. Technical contacts: Charlie Strauss and Ramesh Jha
Capability Enhancement
Prototype Red Sage experiments improve design and diagnostics
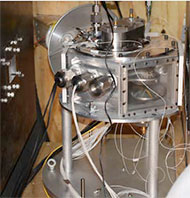
Photo. Silverleaf package installed at the Q-site firing point.
The Red Sage campaign is a series of subcritical dynamic plutonium experiments designed to measure ejecta. Nightshade – the first Red Sage series scheduled for fiscal year 2019 – will measure the amount of ejecta emission into vacuum from a double-shocked plutonium surface. The data are required to develop and calibrate ejecta models used in LANL’s codes to predict device performance.
The project will field a complex suite of diagnostics, including velocimetry, momentum measurements, and radiography, for full diagnosis of the ejecta emission. Several targets will be driven at the same time to maximize data return. This complexity is a major technical risk to the Nightshade subcritical experiment.
A team executed four experiments in the Silverleaf series at the Lab’s Q-site to address this technical risk. The work demonstrated a prototype design of the Nightshade package. This package met the constraints for fielding at the Nevada National Security Site U1a facility. All diagnostics obtained excellent data, and the team successfully fielded broadband laser ranging for the first time. Assembly and data quality improved with each experiment. The work enabled a comparison of radiographic and momentum-transfer ejecta mass-velocity diagnostics on double-shock ejecta packages in geometries representative of the Nightshade in-vessel design.
The experiments satisfied the Level 2 milestone to validate the preliminary Nightshade in-vessel fielding geometry by demonstrating inter-diagnostic agreement with the relevant geometries, standoff distances, and conjugates. As a result of the Silverleaf series, a number of design improvements have been identified for the Nightshade experiments. These improvements cover many aspects of the design, from diagnostic improvements to manufacturing and assembly methods that will result in a more efficient and higher quality experiment.
Reference: “Nightshade Prototype Experiments (Silverleaf),” September 2016; LA-UR-16- 27510. Researchers: R. Gentzlinger (Analytics, Intelligence, and Technology, A), D. Oschwald (Chemistry, C), D. Creveling, D. Doty, S. Gilbertson, R. Guffee, D. Naranjo, S. Rivera, and M. Shinas, (Integrated Weapons Experiments, J); C. Campbell and A. Cartelli (Explosive Science and Shock Physics, M); D. Schmidt (Materials Science and Technology, MST); M. Briggs, W. Buttler, J. Danielson, D. Duke, P. Harding, T. Mangan, R. Manzanares, P. Medina, P. Pazuchanics, J. Payton, D. Sorenson, B. Stone, L. Tabaka, and P. Younk (Physics, P); D. Aragon, E. Aragon, M. Herrera, V. Hesch, T. Martinez, L. Montoya, and J. Olivas (Prototype Fabrication, PF); R. Hall, G. Liechty, and R. Roybal (Weapons Stockpile Modernization, Q); C. Cunico, R. Gonzales, R. Originales, M. Tompkins, and D. Villareal (Weapons Systems Engineering, W); A. Bauer, M. Furlanetto, and C. Hagelberg (X-Theoretical Design, XTD); S. Baker, A. Corridor, E. Daykin, A. Diaz, M. Hanache, B. Lalone, A. Lopez, D. Morgan, D. Phillips, D. Smalley, A. Smith, M. Pena, and J. Stevens (National Security Technologies); C. Bennett, S. Compton, L. Ferranti, A. Lodes, J. Sinibaldi, and P.l Steele (Lawrence Livermore National Laboratory).
NNSA Science Campaign 2 (lead by Michael Furlanetto) funded the work, which supports the Lab’s Nuclear Deterrence mission area and the Nuclear and Particle Futures and Science of Signatures science pillars. Technical contacts: Jeremy Danielson and Amy Bauer
Novel liquid helium technique aids search for a neutron electrical dipole moment
A multi-institutional team at Los Alamos has constructed a device measuring the electrical breakdown of liquid helium, thus demonstrating a novel technique enabling a highly sensitive search for the non-zero electrical dipole moment (EDM) in the neutron. For 60-some-years scientists have been searching for a signature of the neutron EDM, whose effects are influenced by the strength of the applied electric field. By successfully showing that liquid helium can be used to create an electric field much larger than in previous experiments, the team’s technique could aid experiments aimed at explaining the universe’s apparent imbalance of matter and antimatter. The team’s technique will be used during the upcoming hunt for a neutron EDM at Oak Ridge National Laboratory’s Spallation Neutron Source, which leads the search. The DOE Office of Science featured the work as a highlight on its website. An article in Review of Scientific Instruments describes the apparatus’s design, construction, and operational experience, as well as initial results.
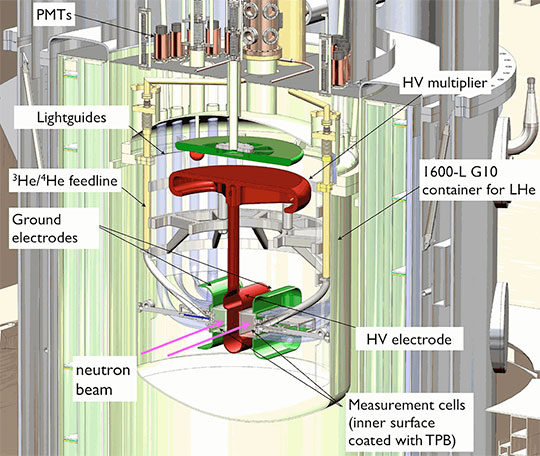
Figure 4. A schematic of the MSHV apparatus.
The team constructed the medium-scale high voltage (MSHV) test apparatus to study electrical breakdown in liquid helium, which can withstand a higher electric field than a vacuum. The results showed DC electrical breakdown at temperatures as low as 0.4 K and at pressures between the saturated vapor pressure and approximately 600 Torr. These results indicate that liquid helium can be used to apply an electric field several times bigger than that of previous neutron EDM experiments.
Reference: “An Apparatus for Studying Electrical Breakdown in Liquid Helium at 0.4 K and Testing Electrode Materials for the Neutron Electric Dipole Moment Experiment at the Spallation Neutron Source,” Review of Scientific Instruments 87, 045113 (2016); doi: 10.1063/1.4946896. Authors: T. M. Ito, J. C. Ramsey, S. M. Clayton, S. A. Currie, M. Makela, Z. Tang, and W. Wei (Subatomic Physics, P-25); W. C. Griffith (former P-25 postdoc researcher, now with the University of Sussex); V. Cianciolo and W. Yao (Oak Ridge National Laboratory); S. E. Williamson and D. H. Beck (University of Illinois); D. Wagner and C. Crawford (University of Kentucky); B. W. Filippone and R. Schmid (California Institute of Technology); and G. M. Seidel (Brown University). The DOE, Office of Science, Office of Nuclear Physics funded the LANL research. The work supports the Lab’s Energy Security mission area and the Nuclear and Particle Futures and Science of Signatures science pillars by developing experimental techniques for cryogenics, high voltages, particle detection, and precision measurements. Technical contact: Takeyasu Ito
Earth and Environmental Sciences
Accounting and risk analysis for CO2 sequestration at enhanced oil recovery sites
Geological carbon sequestration is a leading candidate for permanent storage of carbon dioxide (CO2) or other forms of carbon to mitigate global warming and avoid extreme climate changes. CO2 is also an attractive displacing agent for enhanced oil recovery (CO2-EOR) because it has a relatively low minimum miscibility pressure (i.e., the minimum pressure by which crude oil remains miscible with CO2 at reservoir temperature) in a wide range of crude oils. Because a large portion of the injected CO2 remains in place in depleted reservoirs after CO2-EOR, it is an option for permanently sequestering CO2 with reduced costs.
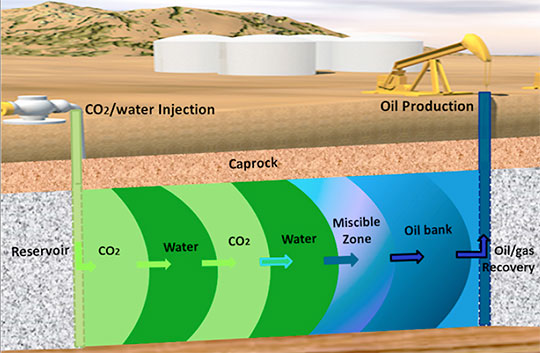
Figure 5. Schematic of the water-alternating-gas (WAG) process.
However, very low viscosity often causes CO2 to break through to production wells, and poor mobility control may leave large areas of the reservoir unswept. To overcome this disadvantage, current CO2-EOR projects alternatively inject gas and water (or brine) as slugs in the water-alternating-gas (WAG) method to control CO2 mobility and flood conformance. WAG can be very effective, but more detailed studies of CO2 interaction with oil, formation water, and heterogeneous sediments are needed to understand the mechanism of CO2 geological sequestration in oil/gas reservoirs and quantitatively evaluate the total amount of CO2 irreversibly stored in reservoirs. LANL scientists and collaborators developed a generic multiscale statistical framework for CO2 accounting and risk analysis in CO2-EOR sites. The journal Environmental Science & Technology published the research.
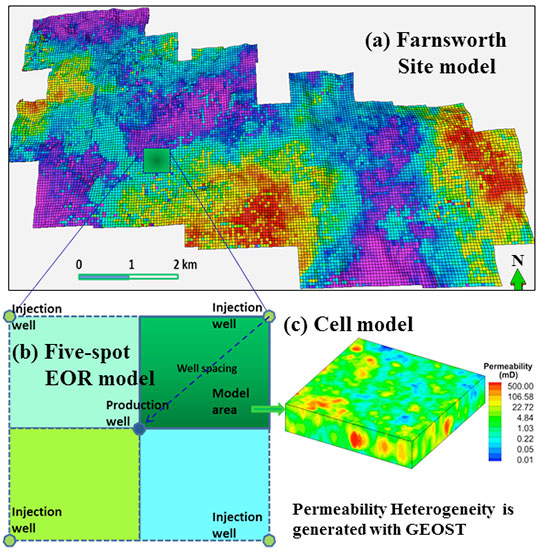
Figure 6. Multi‐scale simulations of the CO2-oil-water flow and transport in the heterogeneous reservoir based on a five‐spot EOR pattern (four injection wells in the corners of a square and a production well in the center). a) Farnsworth site model for porosity. Red color indicates large porosity (0.25) and blue color indicates small porosity (0.03). b) Five-‐spot EOR model. c) Generated permeability heterogeneity in a cell model. GEOST is a LANL-‐ developed statistical modeling tool.
Figure 6 shows the analysis processes of the multi-scale statistical framework. In most CO2-EOR sites such as in the Farnsworth Unit (FWU, Texas) site, the exact values of the reservoir parameters are not well known. However, enough information may be obtained to characterize or define the uncertainty distributions of these parameters. These distributions are used to sample the uncertain parameters and conduct geostatistical-based Monte Carlo simulations.
A set of risk factor metrics is defined to post-process the Monte Carlo simulation results for statistical analysis. The risk factors are expressed as measurable quantities to gain insight into project risk (e.g., environmental and economic risks) without the need to generate a rigorous consequence structure of CO2 injection rate, cumulative CO2 storage, cumulative water injection, cumulative oil/gas production. The FWU site served as an example to study the multiscale statistical approach for CO2 accounting and risk analysis. The results provide insights to understand CO2 storage potential and the corresponding environmental and economic risks of commercial-scale CO2- sequestration in depleted reservoirs.
Reference: “CO2 Accounting and Risk Analysis for CO2 Sequestration at Enhanced Oil Recovery Sites”, Environmental Science & Technology 50, 7546 (2016); doi: 10.1021/acs.est.6b01744. Authors: Zhenxue Dai, Hari Viswanathan, and Richard Middleton (Computational Earth Science, EES-16); Feng Pan, Wei Jia, Ting Xiao, and Brian McPherson (University of Utah – Salt Lake City); William Ampomah, Robert Balch, and Reid Grigg (New Mexico Tech); Changbin Yang (University of Texas – Austin); Si-Yong Lee (Schlumberger Carbon Services); and Mark White (Pacific Northwest National Laboratory).
This work is part of Phase III of the Southwest CO2-EOR/Storage Project, which DOE funds and National Energy Technology Laboratory manages through the Southwest Partnership on Carbon Sequestration. The research supports LANL’s Energy Security mission area and the Information, Science, and Technology science pillar. Technical contacts: Zhenxue Dai and Hari Viswanathan
Engineering Institute
Blind identification of full-field vibration modes from video measurements
Engineering structures (e.g., civil infrastructure and airplanes) usually have complex geometries, material properties, and boundary conditions. When subjected to dynamic loading (e.g., hurricanes, earthquakes, and extreme events such as blast), they can exhibit spatially local, temporally transient, dynamic behaviors and vibrations. Measuring these structural dynamic responses at a high spatial and temporal resolution is essential to characterize the structure’s dynamics and validate the finite element model. However, obtaining high-resolution vibration measurements using traditional techniques is problematic. For example, accelerometers and strain-gauge sensors can only be placed at a limited number of locations due to the costs and the associated deployment and maintenance, thus providing low spatial resolution structural vibration measurements. Laser vibrometers provide high-resolution vibration measurements in a scanning mode, but the sequential measurements are time-consuming for large engineering structures. LANL researchers and collaborators have developed a new approach that overcomes these obstacles. The journal Mechanical Systems and Signal Processing has published the work.
The LANL team and Los Alamos Dynamics Summer School students addressed this challenge by using digital video cameras where every pixel becomes a measurement point. They developed an innovative algorithmic technique that automatically extracts the vibration and dynamics (mode shapes, modal frequencies, and damping ratios) at each pixel on the structure from its video. This enables extremely high-spatial-resolution or full-field vibration measurements and dynamics characterization of the structure. The researchers connected the physical mapping between unsupervised machine learning model (blind source separation) and high-spatialdimensional dynamics model (modal superposition) to enable automated, very efficient implementation. This computational approach extracts full-field high-frequency structure dynamics using low-speed, commercially available off-the-shell digital video cameras to enhance the temporal resolution of their full-field imaging approach.
The team predicts great potential with the full-field imaging technique, which effectively instruments a structure with a spatially dense array of sensors. The significantly more abundant structural measurements and dynamics information extracted from the video enable experimental validation and update the highly refined finite element models of the structure. Digital video cameras are non-contact, relatively low-cost, agile to set up, and provide high spatial (pixel) resolution and simultaneous measurements. Compared with existing digital imaging techniques such as digital image correlation, the video approach does not require installing a speckle pattern or makers on the structures’ surface. The resources required for its deployment are minimal, making it practical for applications in large engineering structures. Moreover, it reduces computation requirements by orders of magnitude compared with existing techniques.
This new full-field imaging method can locate structural defects of a small magnitude and at a resolution that were not possible previously. In lab experiments, the team successfully located, in a pixel’s resolution, a 1/16’’ hole drilled half through the thickness of a 6”x1”x1/8” beam component, which was not visible to the camera. The damage was equivalent to a 3% cross section stiffness reduction. The completely passive approach uses only the video of the structure without any baseline or reference information. They examined the high-spatial-resolution mode shapes of the structure and located damage-induced irregularity in the mode shape, even though such a feature is extremely subtle. The high resolution (more than 100 simultaneous pixel measurement points in a 6” length) and small magnitude of damage has far exceeded the state-of-the-art performance (25% cross-section damage with about 10 accelerometers installed in the vicinity using a priori knowledge). This result opens the possibility of detecting small defects in structures using a low-cost and agile digital camera imaging system.
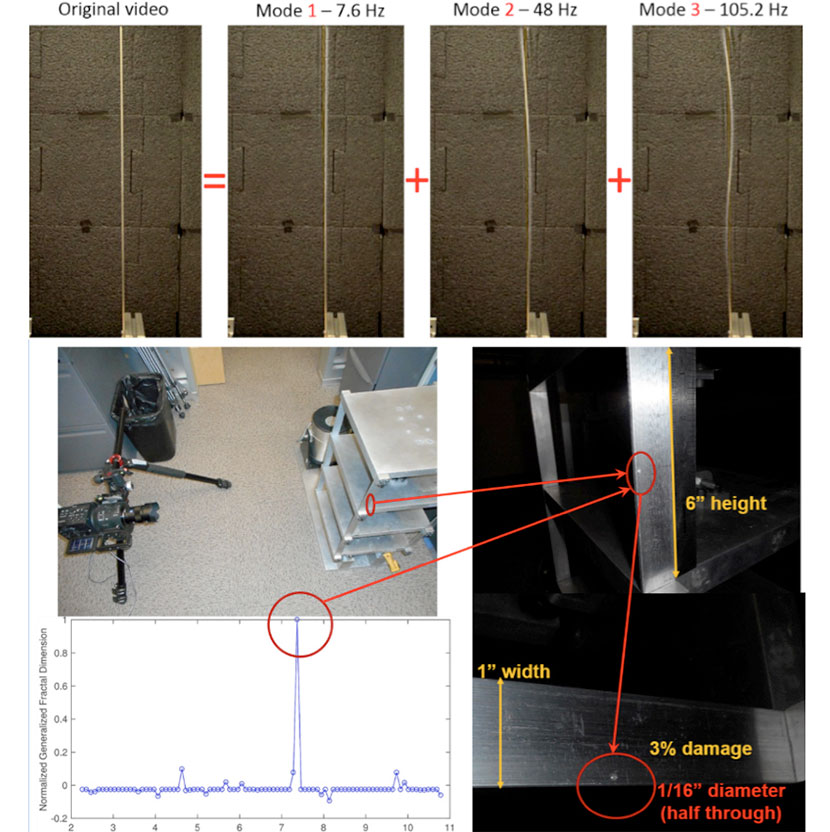
Figure 7. (Top row): The full‐field digital imaging technique applied to an operating cantilever beam excited by a hammer impact. The original video is blindly and autonomously separated into three videos; each contains one individual vibration mode with very high spatial (pixel) resolution. (Bottom left): The fractal dimension curves analyzing the full-field 1st mode shapes blindly extracted from the video measurements of the damaged right top front column of the 3-‐story building structure excited by an impact hammer. Photos show that damage is a hole drilled half way through the thickness equivalent to a 3% cross section stiffness reduction.
Reference: “Blind Identification of Full-Field Vibration Modes from Video Measurements with Phase-Based Video Motion Magnification,” Mechanical Systems and Signal Processing 85, 567 (2017); published online ahead of print doi: 1 0.1016/j.ymssp.2016.08.041. Authors: Yongchao Yang, Charles Farrar, and David Mascareñas (National Security Education Center/Engineering Institute, NSEC/EI); Charles Dorn (University of Wisconsin); Tyler Mancini (University of Texas – Austin); Zachary Talken (Missouri University of Science and Technology); and Garrett Kenyon (Information Sciences, CCS-3). (Supplementary videos: http://www.sciencedirect.com/science/article/pii/S0888327016303272 and https://www.lanl.gov/projects/national-security-education-center/engineering/researchprojects/ blind-modal-id.php)
The Los Alamos National Laboratory Research and Development (LDRD) program sponsored this work via a Director’s funded postdoctoral fellowship for Yongchao Yang and an Early Career Award for David Mascareñas. The Los Alamos Dynamics Summer School has funded the students. The work supports the Lab’s Energy Security and Nuclear Deterrence mission areas and the Science of Signatures science pillar through the ability to detect dynamic response and defects in engineered structures. Technical contacts: Yongchao Yang and David Mascarenas
Materials Physics and Applications
Engineering nanoscale metamaterials using a novel self-assembly approach
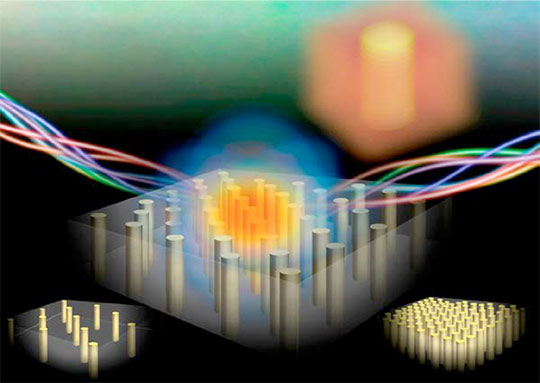
Figure 8. Schema of a self-assembled epitaxial metal vertically aligned nanocomposite (VAN) structure. The schematic demonstrates the concept of metal−oxide VAN structures as nanostructured metamaterials with tunable metallic nanopillar density.
Center for Integrated Nanotechnologies (CINT) users and Quanxi Jia (Center for Integrated Nanotechnologies, MPA-CINT) have demonstrated a new self-assembly approach to fabricate nanoscale metamaterials made of epitaxial metallic nanopillars in oxide matrices. This method permits control over the density, size, and alignment of nanopillars instead of a random distribution of particles in the oxide matrix. This control opens new possibilities for large scale, reliable development of nanoscale photonic materials that enhance light-matter interactions at the nanoscale for novel applications. The study appeared in Nano Letters.
Metamaterials are engineered to have a property that is not found in nature. They are made from assemblies of multiple elements fashioned from composite materials. The materials are usually arranged in repeating patterns, at scales that are smaller than the wavelengths of the phenomena they influence. Metamaterials derive their properties from their designed structures rather than the properties of the base materials. These new materials exhibit exotic optical properties that have potential promising applications, such as super-resolution imaging, cloaking, hyperbolic propagation, and ultrafast phase velocities. Bottom-up template-assisted electroplating and topdown nanofabrication techniques have been examined to create these materials. However, most metamaterials require costly and tedious fabrication techniques with limited paths toward reliable large-scale fabrication. This limits the applicability of the materials for useful applications.
The team developed a one-step self-assembly method as a drastically different approach to fabricate large area nanostructured metamaterials. The researchers used pulsed laser deposition method to fabricate the nanoscale metamaterials, creating films with vertically aligned gold nanopillars (approximately 20 nm in diameter) embedded in various oxide matrices with high epitaxial quality. The density of self-assembled gold nanopillars in the vertically aligned nanocomposite (VAN) films could be easily tailored by controlling the content of gold in the composite target. Three steps can describe the formation mechanism of self-assembled epitaxial metal-oxide VAN structures: 1) adatom (adsorbed atom) diffusion, 2) initial two-phase nucleation, and 3) further growth of the two phases. Gold adatoms diffuse and nucleate as islands to minimize its high surface energy, while metal oxide adatoms nucleate to form the overall matrix via layer-by-layer growth. After the initial nucleation, the gold and metal oxide adatoms further diffuse and grow in the form of gold nanopillars embedded in a metal oxide matrix. Thus, a unique gold nanopillar-oxide matrix nanocomposite structure forms through the film thickness.
Angular dependent and polarization resolved reflectivity measurements supported by full-wave simulations and effective medium theory demonstrated the anisotropic optical properties of the metamaterials. The team’s model predicted exotic properties, such as zero permittivity responses and topological transitions. Compared with other fabrication techniques, this self-assembled onestep epitaxial growth of metal-oxide nano-composites permits control over the density, size, and alignment of nanopillars. The results suggest that self-assembled metal-oxide nanostructures may be a promising new material platform to control and enhance optical response at nanometer scales for a variety of useful applications.
Reference: “Self-Assembled Epitaxial Au-Oxide Vertically Aligned Nanocomposites for Nanoscale Metamaterials,” Nano Letters 16, 3936 (2016); doi: 10.1021/acs.nanolett.6b01575. Authors: Leigang Li, Nicki L. Hogan, Fauzia Khatkhatay, Wenrui Zhang, Jie Jian, Jijie Huang, Qing Su, Meng Fan, Clement Jacob, Jin Li, Xinghang Zhang, Matthew Sheldon, and Haiyan Wang (Texas A&M University); Liuyang Sun, Juan Sebastian Gomez-Diaz, Andrea Alù, and Xiaoqin Li (The University of Texas - Austin); Ping Lu (Sandia National Laboratories); and Quanxi Jia (Center for Integrated Nanotechnologies, MPA-CINT).
The Laboratory Directed Research and Development Program (LDRD) funded the Los Alamos work, which was performed, in part, at the Center for Integrated Nanotechnologies (CINT), an
Materials Science and Technology
First-ever rare-earth free permanent magnets with 20 MGOe energy product
A team of researchers from the University of Minnesota, Los Alamos National Laboratory, and Oak Ridge National Laboratory has prepared the first-ever rare-earth-free-magnet with a magnet performance of 20 MGOe (megagauss-oersted). The stored energy in a magnet is called magnet performance or maximum energy product. The journal Scientific Reports published the research.
Demand for permanent magnets is increasing due to their higher efficiency and smaller size than the electromagnets in motors and generators. The most powerful, durable, and useful permanent magnets contain the rare earth elements neodymium or samarium. However, rare earths present challenges: 1) they are expensive, 2) the extraction process is energy intensive and hazardous for the environment, and 3) a specific region of the world dominates the supply chain. All these pose a major problem when the world depends on rare-earth-based permanent magnets for running motors, generators, sensors, and many other machines and devices.
The group of researchers synthesized a rare-earth-free permanent magnet based on an iron (Fe) and nickel (Ni) α″-Fe16N2 martensite (metastable iron phase) structure. The raw material is abundant and cheap, and the excellent magnetic properties of the material (in theory up to 130 MGOe) had been predicted. However, the material had been very difficult to make previously. The desirable α″-martensite crystal structure is only stable below 214 oC, but a temperature greater than 300 oC is needed to give the material the correct grain microstructure. Therefore, the α″-Fe16N2 martensite microstructure cannot be achieved via traditional annealing.
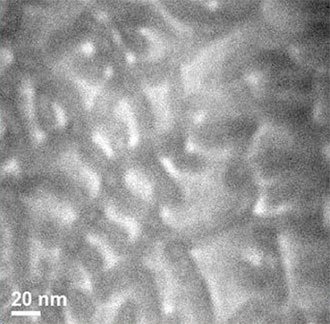
Figure 8. Microstructure of the prepared sample, observed by high resolution transmission electron microscopy (HRTEM). A 5 x 1017/cm2 fluence generates a microstructure with a clear boundary.
The team bonded an iron layer to a silicon wafer. They prepared a 500 nm α″-Fe16N2 foil by first implanting a high fluence of nitrogen ions in a pure iron matrix, followed by a two-step thermal annealing process to engineer the foil for the high energy product. The investigators used the tunable capability of ion implantation fluence and energy to construct a microstructure with grain size of 25–30 nm on the FeN foil sample. Ion implantation is a commonly used technology in the semiconductor industry. The authors suggest that this method of materials processing could be developed for the bulk scale to manufacture the rare-earthfree magnetic material.
This is the first-ever demonstration of energy product of 20 MGOe from a rare-earth-free magnet. In comparison, neodymium and samarium magnets score 10-48 and 16-33 MGOe, respectively. The new material possesses a giant saturation magnetization and a large magnetic coercivity. Magnetic coercivity is a measure of the ability of a material to withstand an external magnetic field without becoming demagnetized. A large magnetic coercivity differentiates permanent (“hard”) magnets from “soft” magnetic materials that cannot support a permanent field. Thus the rare-earth-free-magnet demonstrates excellent properties for a permanent magnet.
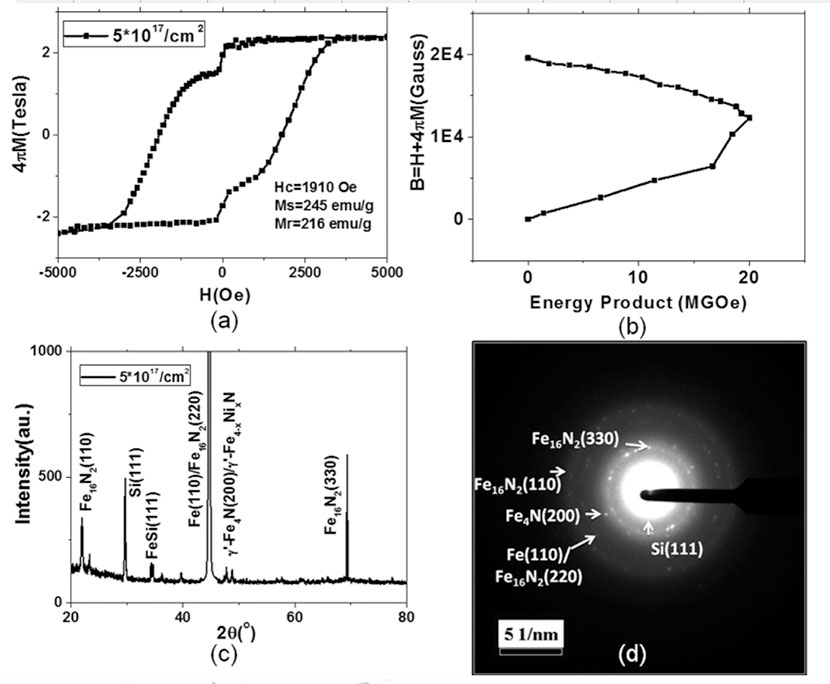
Figure 9. Characterization results of a bulk Fe16N2 free‐standing foil prepared by the nitrogen ion implantation method with 5 × 1017/cm2 fluence (a) In‐plane hysteresis loops for the sample at room temperature, showing Hc = 1910 Oe, Ms = 245 emu/g, Mr = 216 emu/g; (b) The calculated energy product, indicating maximum value 20 MGOe; (c) The X-‐ray diffraction spectrum, showing the Fe16N2 phase generated in the foil; (d) The HRTEM diffraction pattern of FeN sample with 5 × 1017/cm2 fluence, showing Fe16N2, Fe4N/Fe4−xNixN and Fe phases.
Reference: “Synthesis of Fe16N2 compound Free-Standing Foils with 20 MGOe Magnetic Energy Product by Nitrogen Ion-Implantation,” Scientific Reports 6, 25436 (2016); doi: 10.1038/srep25436. Authors: Yanfeng Jiang, Md Al Mehedi, and Jian-Ping Wang (University of Minnesota – Minneapolis); Engang Fu and Yongqiang Wang (Materials Science in Radiation and Dynamics Extremes, MST-8); and Lawrence F. Allard (Oak Ridge National Laboratory).
The Center for Integrated Nanotechnologies (CINT), a DOE Office of Science nanoscience user facility operated by Los Alamos and Sandia national laboratories, sponsored the Los Alamos participation. The work supports LANL’s Energy Security mission area and Materials for the Future science pillar through development of materials for clean energy. Technical contact: Yongqiang Wang
Physics
Ejecta transport studies in gases find high cerium-to-cerium hydride conversion rate
A team from the Los Alamos, National Security Technologies, and Yale University reported an experimental study of reactive and nonreactive ejecta transporting in vacuum and reactive and nonreactive gases that resulted in a first observation of its kind. The researchers developed infrared imaging to quantify the amount of reactive metal fragments that chemically reacted during transport in high pressure and temperature reactive gases. Ejecta form as Richtmyer-Meshkov (RM) unstable growth from perturbations on shocked surfaces. Linear tooling marks left on metals during the machining and fabrication process usually create the surface perturbations, which cause mass ejections of 2-D unstable sheets (or 3- D spikes from divots) as a shockwave reacts and releases at the metalgas interface.
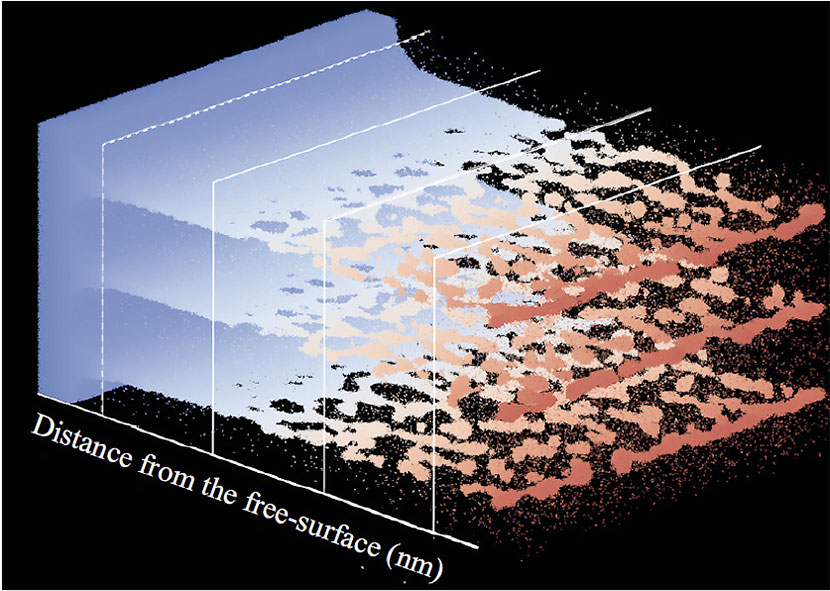
Figure 10. A molecular dynamics simulation of ejecta formation during Richtmyer-Meshkov unstable growth from perturbations on shocked surfaces. During the 2-D ejecta phenomena, the formation of sheets first form holes, then ligaments and particles as the impulse driven RM unstable sheets stretch and self-similarly expand. Credit: O. Durand and L. Soulard (CEA)
Ejecta emissions are relatively large if the surface is melted or the perturbations are large. Less ejecta emit from surfaces that remain solid or are initially mirror-like (diamond finishes). A predictive hydrodynamic capability requires understanding ejecta phenomena, having a capability to measure ejecta masses, sizes, and modeling and hydrodynamically simulating the formation and disposition of ejecta particles in gases.
The team theorized that reactive metal fragments ejected into a reactive gas, such as molecular hydrogen (H2) or deuterium (D2), would fragment even further in situations where they are otherwise hydrodynamically stable in a nonreactive gas (e.g., helium). The investigators suggested that infrared imaging could be used to quantify the amount of reactive metal fragments converted to metal hydride during transport in high pressure and temperature reactive gases.
To test the concept, researchers machined periodic perturbations onto thin cerium (Ce) and zinc (Zn) coupons and then explosively shocked them to eject hot micron-scale fragments into shock compressed reactive and nonreactive gases – deuterium (D2) and helium (He), respectively. The investigators used the National Securities Special Technologies Laboratory to field the experiments. They observed that the radiance temperature of cerium fragments transporting in the shock-compressed 2280 torr D2 was 300° C greater than that of cerium transporting in He in otherwise identical conditions. This result implies that as much as 13 percent of the cerium transporting in deuterium converts to cerium deuteride over a time period of 5.5 μsec subsequent to shock breakout at the cerium surface – a novel observation.
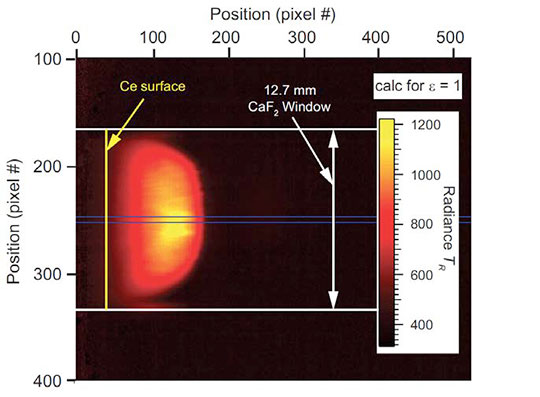
Figure 11. (a) Thermal radiance image of Ce ejecta (traveling left to right), about 5 μs after the ejecta form. The brightest region represents the hottest ejecta. The vertical yellow line denotes the Ce surface.
According to William Buttler (Neutron Science & Technology, P-23), the experimental results could radically alter understanding of ejecta transport and break up. Such a change could make the creation of conversion models for the rapid break up of reactive fragments transporting in a hot, reactive gas into an important element of ejecta transport physics. Understanding these phenomena and being able to measure, model, and hydrodynamically simulate the formation and disposition of ejecta particles in gases are critical for applications in the military, industrial processing, and the field of physics.
Reference: “Ejecta Transport, Break Up and Conversion,” invited to appear in the Ejecta Physics edition of Journal of the Dynamic Behavior of Materials; LA-UR-16-28075 (2016). Authors: W. T. Buttler, A. Llobet, R. Manzanares, and C. L. Pack (P-23); R. K. Schulze and J. C. Cooley (Sigma); M. Grover, B. M. La Lone, G. D. Stevens, W. D. Turley, and L. R. Veeser (National Security Technologies); J. E. Hammerberg and D. G. Sheppard (Materials and Physical Data, XCP-5); J. B. Stone (formerly P-23, now retired); J. I. Martinez and D. W. Schmidt (Engineered Materials, MST-7); J. D. Schwarzkopf (XTD Integrated Design & Assessment, XTD-IDA); and S. K. Lamoreaux (Yale University).
NNSA Science Campaign 1 funds the work, which supports the Lab’s Nuclear Deterrence mission area and the Nuclear and Particle Futures science pillar by furthering understanding of the break up and transportation of ejecta in gases. Technical contact: William Buttler
Sigma
Patent awarded for process to granulate fine radioactive powder
Chris Chen (Sigma Division, Sigma-DO) has developed a novel process for granulating fine powder containing radioactive materials. The technique overcomes the need to add organic binders that can degrade when the powders are needed for nuclear fuel applications. The process received a U.S. Patent.
Wet and dry pressing are common processes to compact fine powder. These methods help prepare products ranging from medicinal pellets to fine-grained technical alumina, magnetic and dielectric ceramics, engineering ceramics, refractories, and nuclear fuel rods. The resulting granulated powder contains deformable granules that are stable under ambient conditions, allowing it to flow more easily into dies. Organic additives such as binders and plasticizers may be added to this granulated powder, where they serve as a deformable medium and may help retain the granule’s shape and structure. Granulated powders could be used for applications in nuclear fuel, but exposure to radiation causes their additives to break down quickly.
In response to this need, Chen developed a novel granulating process that forms a first-greenbody from a fine powder that has radioactive material. (A green-body’s main constituent is weakly bound ceramic material.) High-temperature heat-treating strengthens the weak first green-body, which is then crushed or milled to granulate. Subsequent sieving produces a granulated radioactive powder that may have a desired granule size distribution without the usual organic binders. The product relies on the thermal bonding among the fine powders to maintain its shape and structure, and is still able to flow easily into a die press. The granulated radioactive powder could make up the starting granules to form a second-green-body.
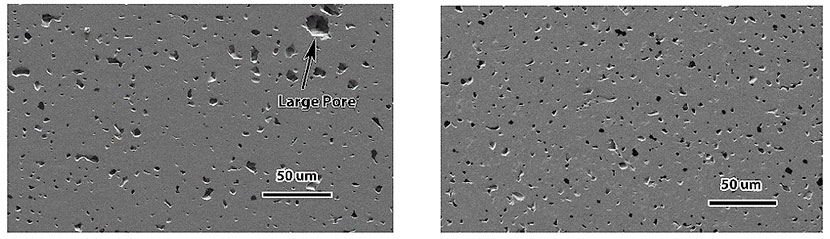
Figure 11. Scanning electron micrographs comparing final sintered microstructures for the green compacts processed with and without the granulation process, respectively. (a) Shows that the microstructure without the granulation has more and larger pores. The microstructure in (b) has fewer, smaller, and more uniform pores.
The weak thermal bonding allows the granulated powder to be deformed easily, similar to the function of organic binder, to give the second-green-body a higher green density and more uniform pore distribution. It can also withstand a radioactive environment and be stored without any degradation. The second-green-body can then be sintered under conditions effective for forming a dense radioactive compact. The patent covers the actinides thorium, protactinium, uranium, neptunium, plutonium, americium, curium, or berkelium and an oxide, nitride, fluoride, chloride, bromide, iodide, sulfide, or combination.
Reference: “Granulation of Fine Powder,” U.S. Patent No. 9,409,825 (August 9, 2016). Inventor: Ching-Fong Chen.
Thor Energy of Norway sponsored the work. The development supports the Laboratory’s Energy Security mission area and Materials for the Future science pillar through creation of a product that could be leveraged for nuclear fuel applications. Technical contact: Chris Chen
Theoretical
First accurate thermodynamic results of the electron component in warm dense matter
A research team, including Travis Sjostrom (Physics and Chemistry of Materials Group, T-1), London Imperial College, and headed by Professor Michael Bonitz (Kiel University), has achieved a major breakthrough in the description of warm dense matter – one of the most active frontiers in plasma physics and material science. Physical Review Letters published the research.
This exotic state of matter is characterized by the simultaneous presence of quantum degeneracy, thermal excitations, and interaction effects. It differs from the usual solid, liquid, gas or plasma states commonly found on earth. The non-trivial interplay of these physical effects has precluded precise theoretical predictions. Warm dense matter exhibits densities up to a thousand times higher than that of normal solids and temperatures exceeding ten thousand times room temperature. Scientists have predicted these extreme conditions to exist within astrophysical objects such as planet cores and white dwarf atmospheres. An improved understanding of warm dense matter will be the key to answer fundamental questions in astrophysics and may even help to determine the age of galaxies.
Warm dense matter conditions can be created under laboratory conditions in laser-excited solids for time scales up to microseconds. This has led to a lot of activity in the field. Researchers need to overcome the challenges arising from this extreme state of matter in order to achieve useful applications. For example, inertial confinement fusion could be a promising source of energy in the future.
Until now, a theoretical description of warm dense matter was only feasible by introducing crude approximations of the underlying physics. Exact simulations have been developed, but were restricted to small model systems containing only a few particles in a limited parameter range.
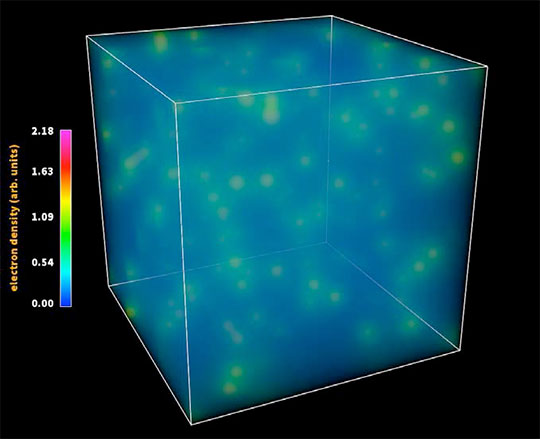
Figure 12. Electron density from a quantum molecular dynamics simulation for a warm dense plasma of deuterium at density 10 g/cc and temperature 10 eV.
Reference: “Ab Initio Quantum Monte Carlo Simulation of the Warm Dense Electron Gas in the Thermodynamic Limit,” Physical Review Letters 117, 156403 (2016); doi: 10.1103/PhysRevLett.117.156403. Authors: Tobias Dornheim, Simon Groth, and Michael Bonitz (Christian-Albrechts-Universität zu Kiel); Travis Sjostrom (Physics and Chemistry of Materials, T-1); Fionn D. Malone and W. M. C. Foulkes (Imperial College London).
NNSA Advanced Simulation and Computing Program/Physics and Engineering Models (ASC/PEM) program funded the LANL work, which supports the Lab’s Nuclear Deterrence mission area and the Information, Science, and Technology and Nuclear and Particle Futures science pillars. Technical contact: Travis Sjostrom