Science Highlights, July 22, 2015
Awards and Recognition
Accelerator Operations and Technology
Vertical Shock Tube experiments drive understanding of unsteady turbulence
Earth and Environmental Sciences
Subsurface Technology and Engineering Crosscut tackles energy grand challenge
Materials Physics and Applications
Doped nanotubes open a new path toward quantum information technologies
Awards and Recognition
Kathy Prestridge named APS’s Woman Physicist of the Month
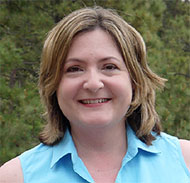
Kathy Prestridge
The American Physical Society (APS) has selected Kathy Prestridge (Neutron Science and Technology, P-23) as the Woman Physicist of the Month for July. The Woman Physicist of the Month award recognizes female physicists who have positively impacted other individuals’ lives and careers.
Prestridge holds a PhD in applied mechanics and engineering science from the University of California – San Diego. She joined the Laboratory in 1998 as a postdoctoral research associate and became a staff member a year later. Prestridge has served as principal investigator, P-23 deputy group leader, project leader, and team leader for the P-23 Extreme Fluids Team.
Prestridge has developed a strong experimental fluids program in the areas of mixing and turbulence. She studies the behavior of materials under high strain conditions, shock-driven instabilities, mixing, and turbulence at high resolution. Prestridge is the principal investigator for the horizontal shock tube, vertical shock tube, and turbulent mixing tunnel experiments conducted at the Laboratory. She applies cutting-edge diagnostics to flows that are high-speed and difficult to measure. New physical insights into shock-driven turbulent mixing and multiphase flows provide important data in support of the Laboratory’s Common Model for mixing and the transport of ejecta. By performing experiments at the small, laboratory scale, her team makes important, novel measurements and performs parametric analyses of difficult mixing and hydrodynamic problems. Researchers incorporate these analyses into models for better predictions of complex flows. The Journal of Fluid Mechanics has highlighted this research on its cover. The NNSA Science Campaigns fund her research in support of predictive science, advanced strategic computing, and modeling efforts.
Prestridge received the Lab’s Postdoctoral Publication Prize in Experimental Science and the Star Award for contributions to the Laboratory and Community. She has won teamwork and mentoring awards, four NNSA Defense Programs Awards of Excellence, and the Saturn Award from Weapons Science Campaign 4 on behalf of the Extreme Fluids Team for producing the first data sets from the Vertical Shock Tube and Turbulent Mixing Tunnel experiments, two new experimental facilities she developed.
Prestridge chairs the American Physical Society’s Committee on the Status of Women in Physics (second term), is co-investigator on a National Science Foundation Grant for the APS’s Professional Skills Development Workshops, and leads the Professional Skills Development Workshop sessions for students and postdocs. She also serves on the Editorial Advisory Board of the journal Experiments in Fluids. Prestridge has mentored 11 postdocs and 21 students during the past decade. Technical contact: Kathy Prestridge
Sherri Bingert receives ASTM International Award of Merit
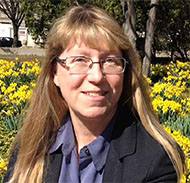
Sherri Bingert
The ASTM International Committee on Metal Powders and Metal Powder Products (B09) has presented the Award of Merit to Sherri Bingert (Weapons Physics, ADX). The Award of Merit includes the title of Fellow and is ASTM’s highest organizational recognition for individual contributions to standards activities.
The citation recognized her for “respected technical expertise, masterful leadership and outstanding contributions to powder metallurgy standards.” She has updated test methods for powder density and flow, revised standards for refractory metals and alloys, and contributed to nomenclature and other characterization methods for metal powders and metal powder products. Bingert holds several officer positions on this Committee, and previously served on the ASTM Committee on Standards. She has also received a Distinguished Service Award from B09. Bingert co-chaired the Technical Program Committee for the 2015 International Conference on Powder Metallurgy and Particulate Materials in San Diego, CA.
Bingert holds a Master of Science degree in metallurgical engineering from the Colorado School of Mines. She specializes in metallurgy and materials science, with an emphasis on powder metallurgy and particulate materials, refractory metals and alloys, precious metals, and alloy and process development. Bingert has spent her entire professional career at LANL. She joined the Lab as a member of the technical staff in 1989 and advanced to various management positions. Bingert has served as program manager of the Laboratory’s Joint Department of Defense/Department of Energy Munitions Technology Development Program, the Dynamic Materials Properties Program, and as deputy group leader for Shock and Detonation Physics. She has received four NNSA Defense Program Team Awards of Excellence and a LANL Large Team Award of Excellence. Bingert currently serves as a senior science advisor to the NNSA Office of Research and Development in Washington, D.C. This is her second NNSA assignment.
ASTM is an international organization that develops standards for manufacturing and materials, products and processes, and systems and services. More than 30,000 of the world’s top technical experts and business professionals are members of the ASTM. Today, some 12,000 ASTM standards are used around the world to improve product quality, enhance safety, facilitate market access and trade, and build consumer confidence. Technical contact: Sherri Bingert
Accelerator Operations and Technology
Vertical Shock Tube experiments drive understanding of unsteady turbulence
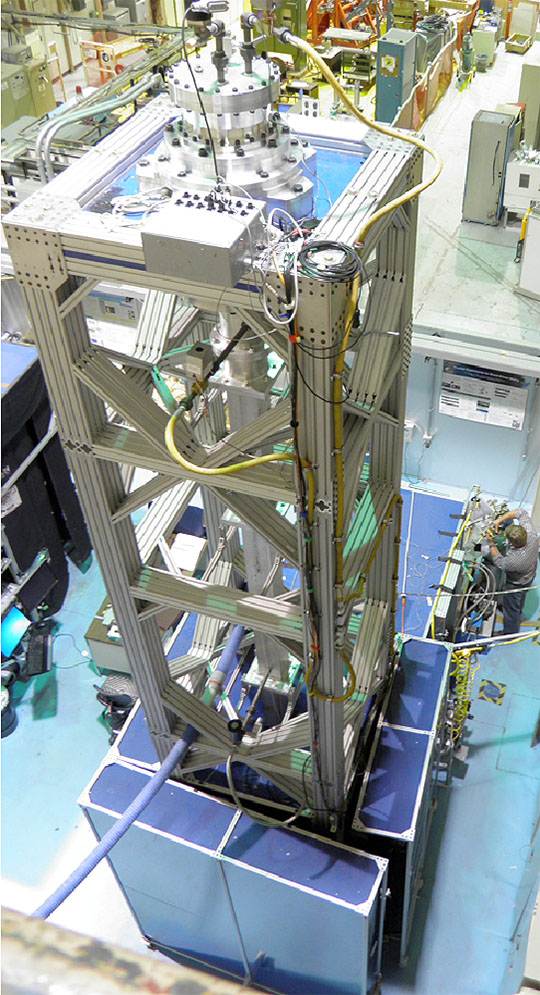
Photo. An aerial view of the Vertical Shock Tube in the Turbulence Laboratory.
The Vertical Shock Tube (VST) experiments in Neutron Science and Technology (P-23) are providing new information about how “atypical” turbulence develops. A team of researchers, including principal investigator Kathy Prestridge (P-23), are using the Vertical Shock Tube to determine the effects that shock waves, initial condition perturbations, and strong density gradients have on turbulent mixing.
The Vertical Shock Tube, located in P-23’s turbulence laboratory, is designed to study shock-driven mixing of two fluids that are struck by a shock wave. The team uses simultaneous velocity and density field diagnostics to measure turbulence quantities in these unsteady flows. Insight into the physics of turbulent mixing helps researchers understand nuclear weapons performance, improves numerical modeling of variable-density turbulence, and provides insight into inertial confinement fusion mixing and the development of supernovae.
Prestridge’s team is making the highest-resolution density and velocity field measurements of shock-driven mixing in the world at the Vertical Shock Tube. Researchers investigate the effects of different initial perturbations on an air-sulfur hexafluoride (SF6) interface that is hit by a shock wave to determine how quickly the flow will mix and become turbulent. New experiments at a shock Mach number of 1.3 (shock traveling at 1.3 times the speed of sound) have revealed that initial conditions with different modes produce different mixing characteristics as the flow evolves. Figure 9 depicts instantaneous mixing fields for three different initial condition experiments. The results demonstrate that the more length scales in the initial perturbations, the more complex the mixing interface after shock. These initial experiments show that the mixing region is not uniform for any of the initial condition types, and many mixing quantities vary throughout the mixing region. Modeling this mixing of two gases is much more complex than single gas turbulence modeling.
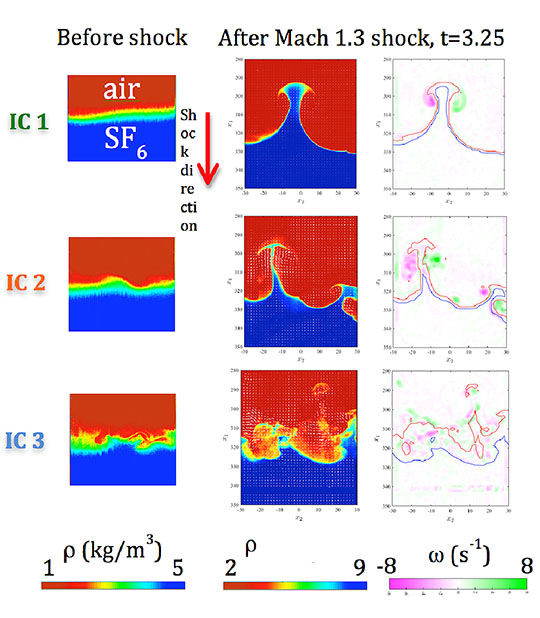
Figure 9. Three different initial condition Vertical Shock Tube experiments at Mach 1.3. (First column): density fields for the three initial condition types, increasing in interface complexity in each row. (Second column): density and velocity fields of the shocked interface 3.25 ms after the shock crosses the initial condition. (Third column): vorticity field with the edges of the mixing layer marked with red and blue lines along the 25% and 75% density contours.
The work is an example of Science on the Roadmap to MaRIE, the Laboratory’s proposed experimental facility for Matter-Radiation Interactions in Extremes. MaRIE’s multi-probe diagnostic hall would enable measurements of density and velocity at the micron frontier under extreme shock loading conditions. The findings could help researchers understand compressibility and other effects in turbulent mixing.
Prestridge presented the recent experimental results at the American Physical Society’s 19th Biennial Conference on Shock Compression of Condensed Matter in Tampa, FL. Researchers include: Kathy Prestridge, Ricardo Mejia-Alvarez, Brandon Wilson, and Adam Martinez (P-23).
NNSA Science Campaigns (John Scott, LANL program manager) fund this work. The research supports the Lab’s Nuclear Deterrence mission area and the Laboratory’s Nuclear and Particle Futures science pillar by providing insight into shock-driven turbulent mixing. Technical contact: Kathy Prestridge
Bioscience
Study of the Burkholderia pseudomallei genome examines differential virulence
Infection by the Gram-negative pathogen Burkholderia pseudomallei results in the disease melioidosis, acquired from the environment in parts of southeast Asia and northern Australia. Clinical symptoms of melioidosis range from acute (fever, pneumonia, septicemia, and localized infection) to chronic (abscesses in organs and tissues), and persistent infections in humans are difficult to cure. Understanding the basic biology and genomics of B. pseudomallei is imperative for the development of new vaccines and therapeutic interventions. The pathogenesis of B. pseudomallei has been challenging to understand due to the extensive natural diversity of its genome.
This formidable task is becoming more tractable due to the increasing number of B. pseudomallei genomes that are being sequenced and compared. LANL scientists and collaborators compared three different B. pseudomallei genomes to understand virulence. PLoS ONE published their results.
Bacterial pathogens have evolved strategies to alter their lifestyle depending on whether they are in their natural environment or infecting a host, shifting resources from normal cell functions to the production of virulence factors, and altering metabolism to take advantage of the nutrients provided by host cells to facilitate survival and growth. The Los Alamos team hypothesized that differences in virulence in B. pseudomallei may be associated with variations in metabolic and regulatory capabilities among strains. The intracellular life cycle and adaptation of a pathogen to the host cell environment depends on the expression of virulence factors, which is controlled by regulatory elements, and may be affected by the metabolic state of the pathogen.
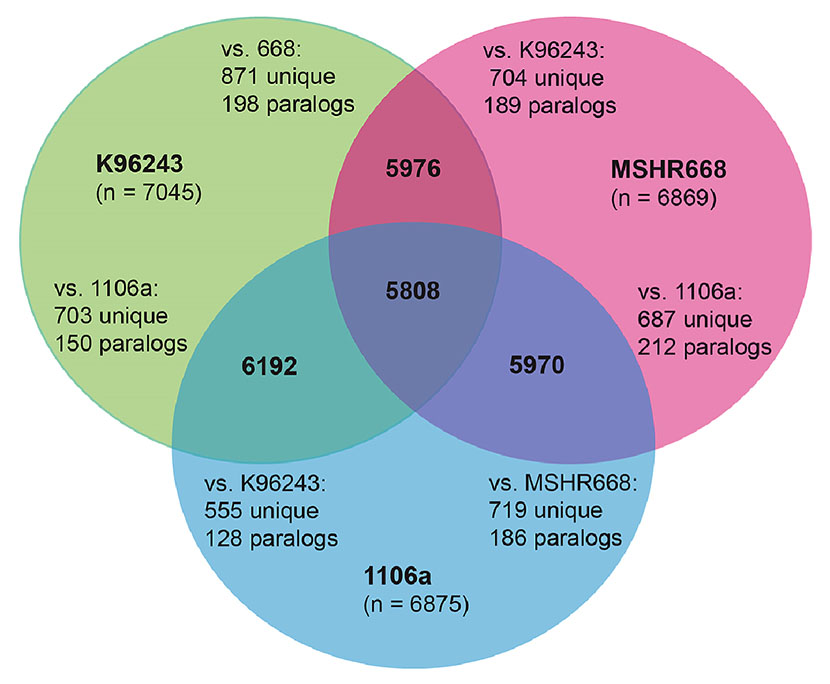
Figure 1. Venn diagram illustrating the numbers of protein coding sequences (CDS) shared by the genomes of B. pseudomallei strains K96243, MSHR668 and 1106a, determined by a bidirectional best BLAST hits analysis. The number of CDS unique to each genome in each pairwise comparison and the number of putative paralogs are shown. Paralogs are created by duplication within a genome. The total number of CDS present in each genome is given below the genome name.
The team compared three B. pseudomallei genomes, from strains MSHR668, K96243 and 1106a, to identify features that might explain why MSHR668 is more virulent than K96243 and 1106a in a mouse model of B. pseudomallei infection. Their analyses focused on metabolic, virulence and regulatory genes that were present in MSHR668 but absent from the other two strains. They also found features present in K96243 and 1106a but absent from MSHR668, and identified genomic differences that may contribute to variations in virulence noted among the three B. pseudomallei isolates. The researchers concluded that the observed differences in transcriptional regulatory genes might contribute to the differential virulence found in this study.
Comparative genomic approaches such as those the authors described could be a key first step in understanding the roles of various bacterial factors in virulence. Further examination of metabolic networks, virulence, and regulation shows promise for examining the effects of B. pseudomallei on host cell metabolism and would lay a foundation for future prediction of the virulence of emerging strains. A better understanding of what constitutes a fully virulent Burkholderia isolate may enable better diagnostic and medical countermeasure strategies.
Reference: “Interrogation of the Burkholderia pseudomallei Genome to Address Differential Virulence among Isolates,” PLoS ONE 9 (12): e115951 (2014); doi: 10.1371/journal.pone.
0115951. Authors include: Jean F. Challacombe (Biosecurity and Public Health, B-10), Chris J. Stubben (Bioenergy and Biome Sciences, B-11), Christopher P. Klimko, Susan L. Welkos, Steven J. Kern, Joel A. Bozue, Patricia L. Worsham, and Christopher K. Cote (US Army Medical Research Institute of Infectious Diseases, Fort Detrick, MD); and Daniel N. Wolfe [Defense Threat Reduction Agency (DTRA), Fort Belvoir, VA].
Department of Defense-Defense Threat Reduction Agency funded the work. The research supports the Lab’s Global Security mission area and the Science of Signatures science pillar through the development of methods to investigate virulence factors in pathogenic organisms. Technical contact: Jean Challacombe
Chemistry
Characterization of products from the hydrolysis of uranium hexafluoride
Uranium hexafluoride (UF6) is a ubiquitous material in various types of nuclear energy, weapons, and research applications. Understanding how hydrolysis affects its chemical speciation and morphology is an important step in predicting both the dispersion of released material and the health consequences of inhaling air-borne particles. The Journal of Fluorine Chemistry will publish work from researchers in Chemistry, Materials Science and Technology, and Materials Physics and Applications divisions that sheds light on this technical issue.
Although UF6 is inert to hydrolysis under dry air, it is quite reactive with water to form the uranium oxy-fluoride material (UO2F2). Theoretical work suggests that the hydrolysis reaction proceeds via a number of intermediates, including UOF4, a product of partial hydrolysis of UF6. The UOF4 intermediate has been detected by Fourier Transform – Infrared Spectroscopy following hydrolysis of UF6 conducted in an argon matrix, a potential trap for reaction intermediates, or in solid films of UF6/H2O mixtures prepared at 12 K and slowly annealed to 242 K. However, UOF4 has not been measured directly during hydrolysis of UF6 in the presence of either excess or substoichiometric water concentrations.
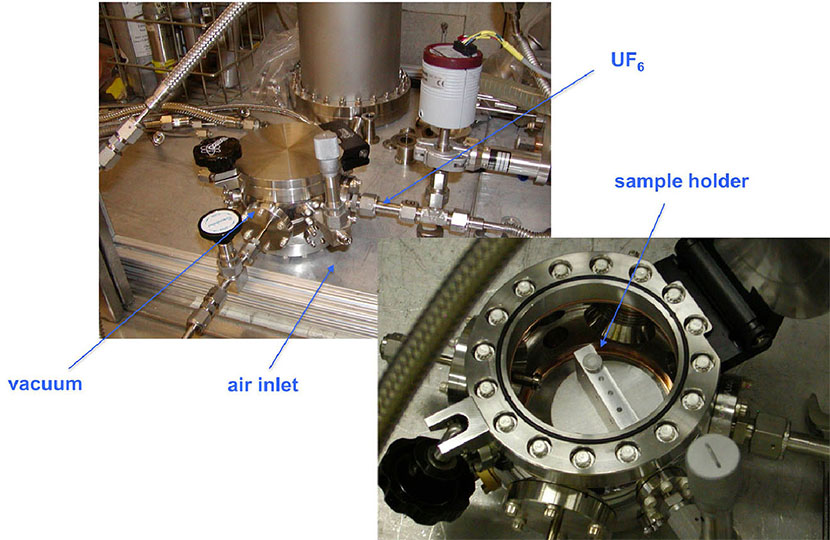
Figure 2. The reaction chamber for the UF6.
The LANL team used a different approach than that reported in previous research to examine the hydrolysis reaction. The Lab scientists produced uranium oxy-fluoride material following introduction of ambient atmosphere into the UF6 vapor under reduced pressure. This route could be possible as a result of a fracture in a UF6 storage containment that would permit air to leak into the vessel such that the air is substoichiometric. In another potential scenario, a large environmental release of UF6 could induce a hypoxic environment on the interior of the reaction cloud, favoring partial hydrolysis of UF6. The researchers observed that different morphologies of reaction products formed on carbon tape versus aluminum substrates. They also stored the deposited material under controlled conditions of temperature and relative humidity to evaluate changes in morphologies and chemical speciation over time. These analyses revealed that the ratio of H2O to UF6, depository substrate composition, and storage conditions over time must be considered in evaluation of the chemical speciation, morphologic texture, and particle size of UF6 hydrolysis products.
Effective strategies for predicting the physical and chemical stabilities of materials, evaluating the dispersive properties of airborne particles, managing the fate of potential contamination, and assessing the health consequences of inhaled contaminants depend upon the ability to characterize physical and chemical transformations of products as a function of storage conductions and time. This new method to conduct water deficient hydrolysis of UF6 in the gas phase could enable such characterizations.
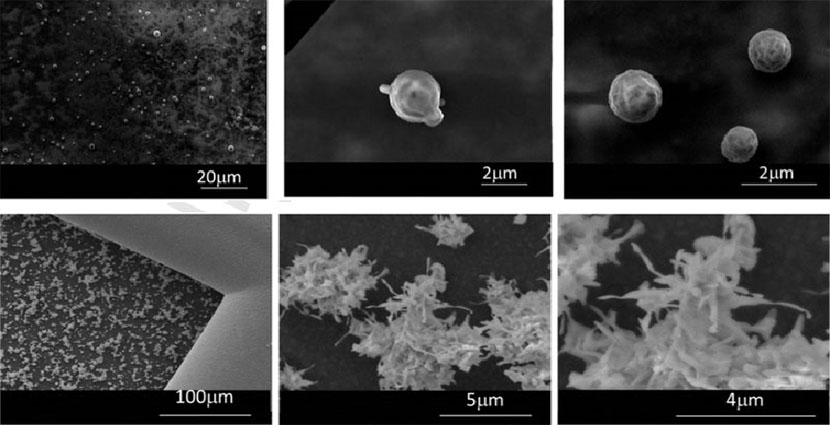
Figure 3. Scanning electron microscope image of material produced under different conditions. Note the morphological changes produced from the differing conditions. (Top): 55 torr of UF6 hydrolyzed under 19% relative humidity and deposited onto carbon tape. (Bottom): 50 torr of UF6 hydrolyzed under 17% relative humidity and deposited onto carbon tape.
Reference: “Morphologic and Chemical Characterization of Products from Hydrolysis of UF6,”
The Laboratory Directed Research and Development (LDRD) Program Office – Reserve funded the work. The research supports the Lab’s mission areas that use actinides and the Science of Signatures and Materials for the Future science pillars. Technical contact: Marianne Wilkerson
Earth and Environmental Sciences
Subsurface Technology and Engineering Crosscut tackles energy grand challenge
Subsurface energy in the form of natural gas, petroleum, coal, and geothermal resources supplies most of the world’s energy and accounts for nearly 80% of the energy used the United States. The subsurface also offers great potential for storage of carbon dioxide (CO2), nuclear waste, and energy (e.g., via compressed air). Harnessing these resources will require technologies with significantly better efficiency and predictability. For example, although unlocking shale gas through fracturing has transformed the global economy, current technologies leave more than 90% of the gas in the reservoir. Therefore, new technologies must maintain long-term flow through fracture networks. In contrast, CO2 must be stored permanently to be impactful. The shale seal must inhibit any flow (including through fractures). An advanced understanding of subsurface and fracture control is critical for many future subsurface energy-related needs. However, despite recent successes and advancements in new extraction methods, significant challenges remain for efficient and environmentally sustainable development of the subsurface. For this reason, the DOE launched the Subsurface Technology and Engineering RD&D (SubTER) Crosscut, a coordinated and highly collaborative initiative working to address subsurface technical challenges that are common to many DOE program areas. By addressing common subsurface challenges such as accessing, engineering, and monitoring, the SubTER Crosscut aims to fulfill societal energy needs as well as administration priorities.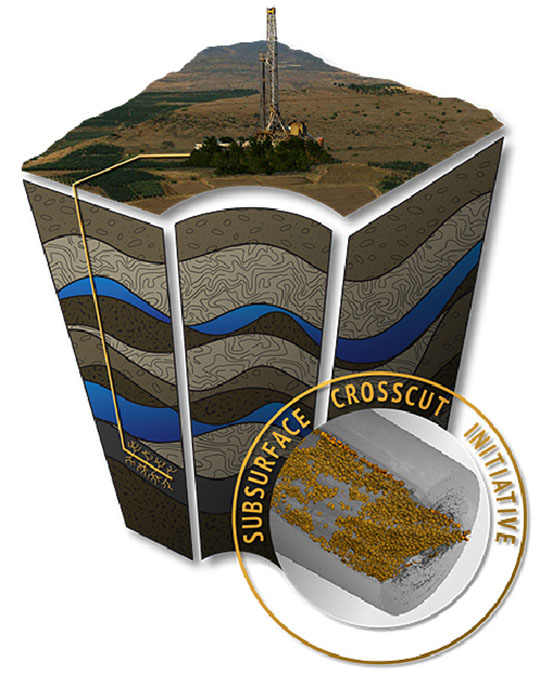
Figure 4. The SubTER Crosscut provides an integrated platform across multiple subsurface interests to address challenges that are associated with the use of the subsurface for energy extraction and storage purposes. Understanding the subsurface geology, fracture network, and fluid flow is essential for this program.
In August 2014, the DOE Office of Energy Efficiency and Renewable Energy (EERE)-Geothermal Technologies Office and the Office of Fossil Energy initiated work on SubTER by awarding approximately $1.6 M to nine national laboratory teams (two of which were from LANL). Multi-lab teams submitted follow-on proposals in early 2015. Los Alamos researchers led two successful proposals and were part of three led by other labs, with a total LANL budget of approximately $1.6 M. Each of these multi-year projects will eventually feed into broader SubTER efforts, which are anticipated to launch in earnest in FY 16.
A federal team from seven program offices (representing geothermal energy, carbon sequestration, oil/gas, nuclear energy, environmental management, energy policy, and science) leads DOE’s
SubTER initiative. This federal team engaged the national labs to identify key challenges and an accompanying technical plan to address them. At the request of DOE, Melissa Fox (Applied Energy,
SPO-AE) has represented Los Alamos on the national-lab executive committee (along with representatives from three other labs). Rajesh Pawar (Computational Earth Science, EES-16 and SPO-AE) has led a multi-lab team in the development of the long-term technical plan for one of SubTER’s four central pillars.
Los Alamos scientists lead two projects that support essential SubTER pillars. The first project – “Evaluating the State of Stress Away from the Borehole” – is led by David Coblentz (Geophysics, EES17) and supports the Subsurface Stress and Induced Seismicity SubTER pillar. Coblentz, Monica Maceira, Paul Johnson, and Andrew Delorey (EES-17); and Satish Karra (EES-16) are extending a LANL-developed inversion model to extract the state of stress from conventional seismic imaging data and gravity surveys and to couple this geophysical information with simulation of flow on discrete fracture networks. The goal is to map changes to the state of stress due to fluid injection in a reservoir.
The second LANL-led project – “Development of Novel 3D Acoustic Borehole Integrity Monitoring System” – is led by Cristian Pantea (Materials Synthesis and Integrated Devices, MPA-11) and supports the Intelligent Wellbores SubTER pillar. For this project, Pantea, Dipen Sinha (MPA-11), and Lianjie Huang (EES-17) are developing a novel wellbore imaging tool based on an acoustic source that provides directional, low but broad frequency range in conjunction with a highly collimated beam. The project aims to produce 3D acoustic images of the environment outside of the borehole, 360° around the borehole axis, a region that is critical both to wellbore integrity and to production from a fractured reservoir. Pantea and Sinha also provide scientific expertise to an effort led by researchers at Oak Ridge National Laboratory that investigates an alternative acoustic approach to image the region outside the wellbore.
In addition, LANL researchers support two projects led by other national labs. Joanne Wendelberger (Statistical Sciences, CCS-6) and James Ahrens (Applied Computer Science, CCS-7) are using LANL strengths in uncertainty analysis and computational methods with large data sets to work with a team led by the National Energy Technology Laboratory. The team plans to develop a probabilistic approach to assess potential for induced seismicity impacts due to fluid injection. Charlotte Rowe (EES-17) and Elena Guardincerri, Christopher Morris, and Matthew Durham (Subatomic Physics, P-25) are using LANL muon detector technology and strengths inversion modeling to work with a team led by the Pacific Northwest National Laboratory. The project aims to develop a down-hole muon detector and a joint-inversion methodology for imaging fluid flow in the subsurface.
The SubTER Crosscut will ultimately enhance efficient and safe use of the subsurface for a growing range of uses, including energy production, CO2 storage, nuclear waste disposal, and wastewater injection while promoting safe environmental management practices and meeting administration goals that enhance national security and fuel economic growth.
DOE EERE-Geothermal Technologies Office and the Office of Fossil Energy (Melissa Fox, LANL Program Director and George Guthrie, LANL Program Manager) fund the research. The work supports the Lab’s Energy Security mission area and the Science of Signatures science pillar by developing of the means to extract subsurface energy sources and to sequester greenhouse gas. Technical contacts: David Coblentz and Christian Pantea
Materials Physics and Applications
Doped nanotubes open a new path toward quantum information technologies
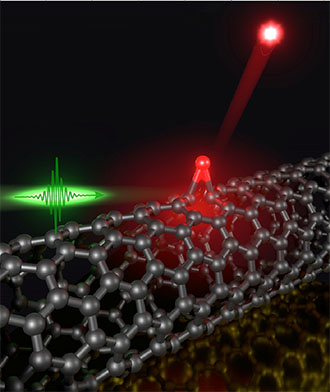
Figure 5. A solitary oxygen dopant (red sphere) covalently attached to the sidewall of the carbon nanotube (gray) can generate single photons (red) at room temperature when excited by laser pulses (green).
In optical communication, critical information ranging from a credit card number to national security data is transmitted in streams of laser pulses. However, one can steal the information transmitted in this manner by splitting out a few photons of the laser pulse. This type of eavesdropping could be prevented by encoding bits of information on quantum mechanical states (e.g. polarization state) of single photons. Realization of such a quantum communication scheme would be a lot easier if the stream of single photons could be generated by simply making a reading lamp dimmer. However, photons emitted from lamps and lasers are distributed randomly in time; therefore “simultaneous” emission of two or more photons is always possible no matter how much they are attenuated.
True single photon generation requires an isolated quantum mechanical two-level system that can emit only one photon in one excitation-emission cycle. In the past decade, atomic-like quantum states of individual atoms, ions and molecules as well as artificial nanoscale materials such as quantum dots, quantum wires, and nitrogen vacancy centers in diamonds have been explored for their potential in single photon generation. However, none of these systems have emerged as the ideal candidate meeting all technological requirements critical for implementation of quantum communication. These requirements include the ability to generate single photons in the 1.3 - 1.5 µm telecommunication wavelength range at room temperature, and compatibility with silicon microfabrication technology to enable electrical stimulation and integration of other electronic and photonic network components. Carbon nanotubes have the potential to meet all these needs. However, earlier studies revealed that nanotubes were capable of single photon emission only at cryogenic temperature, with inefficient emission also showing strong fluctuations and degradation. Therefore, researchers considered nanotubes to be less promising as materials for single photon generation.
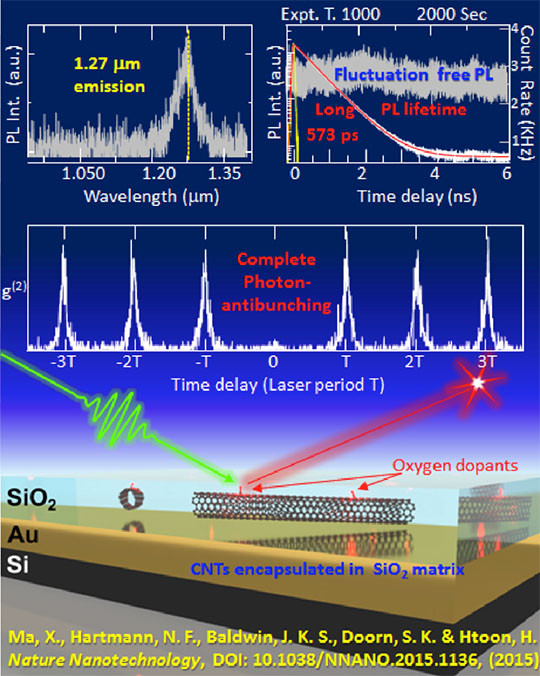
Figure 6. (Top) Left: Optical spectroscopic data of individual dopant states shows 1.27 µm photoluminescence emission. Right: Single exponential photoluminescence decay with 573 ps long lifetime (white and red curves) and fluctuation-free photoluminescence emission (gray trace). (Middle): Second order photon correlation function provides evidence of single photon generation. (Bottom): Schematic illustration of carbon nanotube and oxygen dopant states encapsulated in SiO2 matrix.
In contrast to this belief, researchers led by Han Htoon and Stephen Doorn (Center for Integrated Nanotechnologies, MPA-CINT) reported in Nature Nanotechnology that incorporation of pristine carbon nanotubes into a silicon dioxide (SiO2) matrix could lead to incorporation of solitary oxygen dopant states capable of fluctuation-free, room temperature single photon emission in the 1100 - 1300 nm wavelength range. The team investigated the effects of temperature on photoluminescence emission efficiencies, fluctuations, and decay dynamics of the dopant states to determine the conditions most suitable for the observation of single-photon emission. In principle, the emission could be tuned to 1500 nm via doping of smaller band-gap single-walled carbon nanotubes. This is a distinct advantage compared with diamond nitrogen vacancy centers, in which single photon emission is possible for a few discrete wavelengths shorter than 1 µm.
The oxygen-doped nanotubes can be encapsulated in a SiO2 layer deposited on a Si wafer, presenting an opportunity to apply well-established micro-electronic fabrication technologies for the development of electrically driven single photon sources and integration of these sources into quantum photonic devices and networks. The team has demonstrated oxygen doped carbon nanotubes as a viable system for development of single photon sources. Beyond implementation of quantum communication technologies, nanotube-based single photon sources could enable transformative quantum technologies including ultra-sensitive absorption measurements, sub-diffraction imaging, and linear quantum computing. The work has potential for photonic, plasmonic, optoelectronic and quantum information science applications. It is a significant advance for carbon nanotube optics and might motivate experimental and theoretical studies of new types of covalent dopants with quantum optical and spin properties that enable spintronic and quantum information processing functionalities.
Reference: “Room-temperature Single-photon Generation from Solitary Dopants of Carbon Nanotubes,” Nature Nanotechnology (2015) published online ahead of print; doi: 10.1038/nnano.2015.136. Researchers include: Xuedan Ma, Nicolai F. Hartmann, Jon K. S. Baldwin, Stephen K. Doorn, and Han Htoon (Center for Integrated Nanotechnologies, MPA-CINT).
The Laboratory Directed Research and Development (LDRD) program funded the work, which was performed at the Center for Integrated Nanotechnologies (CINT), a DOE Office of Basic Energy Sciences user facility. The research supports the Lab’s Global Security mission area and the Materials for the Future science pillar via the development of materials for secure communication transmission. Technical contacts: Han Htoon and Stephen Doorn
Materials Science and Technology
Z Machine cylindrical compression experiments on depleted uranium
Researchers executed the first containment experiment (Shot #2824) using depleted uranium in a thin walled cylindrical geometry at Sandia National Laboratories’ Z machine. The team developed a new experimental geometry to acquire quasi-isentropic (nearly constant entropy) compression data at significantly higher peak pressures than previously achievable.
LANL, General Atomics, and Sandia National Laboratories (SNL) collaboratively designed and fabricated the depleted uranium target (Figure 7). The extreme length to diameter aspect ratio required the depleted uranium blanks to be formed by electric discharge machining at Los Alamos. General Atomics precision machined the depleted uranium blanks to final specification and fabricated the target. Key to the success of these experiments was fine-grained uranium, which mattered due to the constrained geometry of these shots. The 15-mm-long cylindrical target was composed of an inner liner made of depleted uranium (inner radius 1.75 mm, 250 μm wall thickness), and an outer liner (the pusher) made of aluminum (inner radius 2.0 mm, 1200 μm wall thickness) that contained the electrical current and magnetic field throughout the implosion.
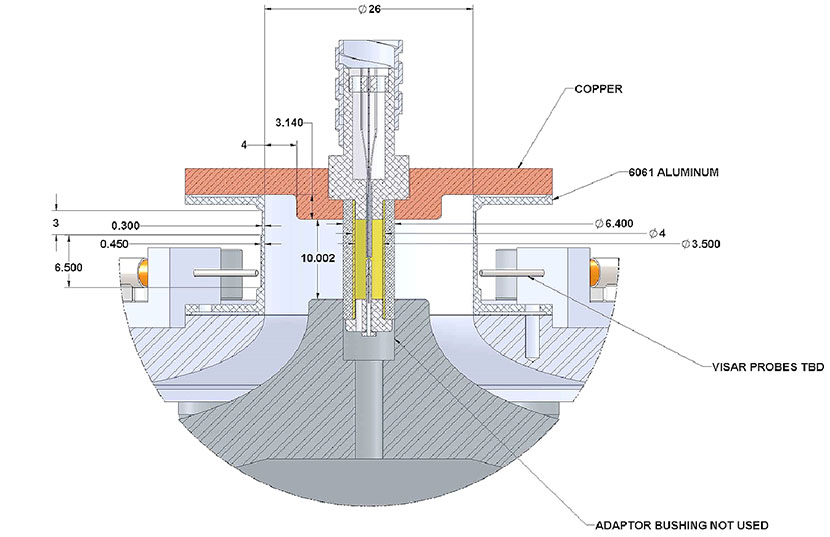
Figure 7. Cutaway cross-section image developed during the depleted uranium target design project phase depicts the experimental configuration of the target assembly. The platform has cylindrical symmetry. The crosshatched gold region (image center) represents the depleted uranium target. The crosshatched gray region that borders it represents the aluminum liner or “pusher”. (All dimensions are in mm.)
The diagnostics included six internal photon Doppler velocimetry (PDV) probes to measure the response of the depleted uranium inner surface to the pressure drive, six Velocity Interferometer System for Any Reflector (VISAR) probes, and two PDV probes that measure the velocity of the external surface of the aluminum anode can. All internal PDV probes returned excellent data. The depleted uranium liner was tracked to the maximum possible velocity and was quasi-isentropically compressed to the peak pressure predicted via magnetohydrodynamics simulation. Radiologic containment during dynamic actinide (i.e., uranium, plutonium, etc.) experiments on the Z machine is dependent on a set of explosively driven ultrafast closure valves. As predicted, Shot #2824 contained the uranium in the chamber.
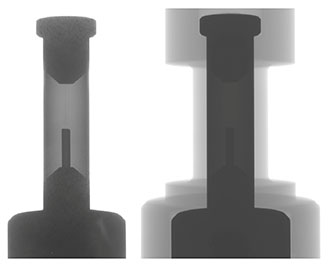
Figure 8. Radiographic images of the target assembly taken at two different contrast or intensity levels. (Left): Image showing semi-transparent depleted uranium thin walled cylinder. Fiber optic probe for PDV diagnostics can be seen through target center. (Right): Image showing semi-transparent aluminum liner or “pusher” with depleted uranium thin walled cylinder appearing as fully dense solid.
LANL and SNL have collaborated on actinide dynamic experiments for over 10 years. This new experimental configuration will facilitate the exploration of actinides behavior to significantly higher pressures and densities. The kind of data obtained for the validation equation of state and other physics performance properties is an example of Science on the Roadmap to MaRIE (Matter-Radiation Interactions in Extremes), the Laboratory’s proposed experimental facility for control of time-dependent material performance. Similar studies could be performed at unprecedented scales using MaRIE’s advanced capabilities.
The LANL team includes: Franz Freibert (Nuclear Materials Science, MST-16), Nenad Velisavljevic (Shock and Detonation Physics, M-9), and David Alexander (Metallurgy, MST-6). NNSA Defense Programs funded the research, which supports the Lab’s Materials for the Future science pillar.Technical contact: Franz Freibert
Physics
Automatic alignment of multiple magnets into a Halbach cylinder
Applied Modern Physics (P-21) researchers have developed a new, simpler technique to assemble a Halbach cylinder, a device that is used in a variety of applications including nuclear magnetic resonance (NMR), magnetic resonance imaging (MRI), magnetic refrigeration, magnetic separation, motors/generators, and charged particle manipulations. The Halbach cylinder is a powerful permanent magnet circuit design that creates a strong, uniform magnetic field distribution inside while minimizing the field outside the cylinder. The new technique replaces a complex, manual fabrication method that has been used since the invention of the Halbach cylinder in the 1980s. The Journal of Magnetism and Magnetic Materials published the new development.
An “ideal” Halbach cylinder is a ring magnet where polarization direction varies continuously along the circumference such that the magnetic flux increases inside, and reduces or cancels on the outside. In practice, it may not be possible to magnetize a hard magnetic ring in a manner where the polarization direction can be varied continuously. Therefore, typical Halbach cylinders have been fabricated manually using discrete permanent magnets, each with its own magnetization direction to approximate the Halbach distribution. The strength and homogeneity from a Halbach design depend on the accuracy and alignment of the constituent magnets. In the manual method, researchers aligned multiple magnets in specific orientations in the presence of strong magnet–magnet interactions and against their natural alignment tendency. Assembly required significant force and specialized tools.
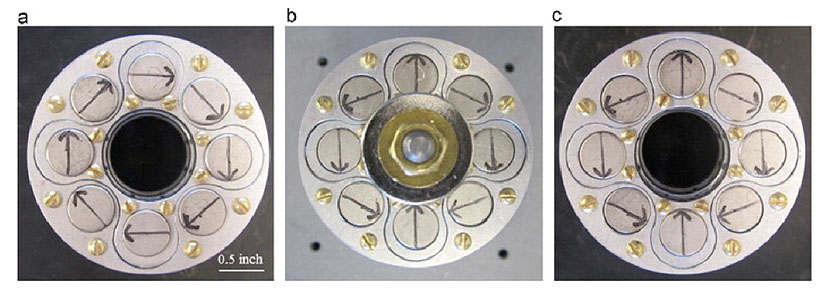
Photos. The different stages of the one-step automatic alignment: (a) natural orientation of the magnets, (b) automatic alignment of the eight section magnets under the influence of the anchor magnet, and (c) the complete prototype with the anchor magnet removed.
Pulak Nath (P-21) led a team that developed a different approach to align the multiple permanent magnets automatically. The scientists used 3D Finite Element modeling to evaluate the extent to which automatic assembly can approximate a Halbach cylinder. The researchers utilized magnetic field distribution from a diametrically magnetized cylindrical magnet where the direction of the magnetic field varies continuously along the circumference, mimicking the distribution in an “ideal” Halbach ring. The team built holders in which multiple cylindrical permanent magnets (identified as sections) can be inserted. Each magnet, located along the circumference of the intended Halbach ring, can rotate freely about its axis. The holder also contains a center slot to insert a diametrically magnetized cylindrical magnet, or “anchor.” Before anchor insertion, the sections are aligned according to their natural interaction. However, when the anchor is inserted, all section magnets simultaneously and automatically rotate into positions forming a Halbach assembly. These magnets can then be secured using glue or set screws. The anchor is removed to obtain the final Halbach assembly. The team also identified a “two-step” approach for applications that require a combination of high magnetic flux density and homogeneity of the fields. The automatic alignment of either approach eliminates the complexity of manual alignment. These assembly methods simplify the fabrication process even for workers inexperienced with permanent magnet fabrication. Thus, automatic assembly can replace traditional permanent magnetic circuits for a broad range of applications.
Reference: “Automatic Alignment of Multiple Magnets into Halbach Cylinders,” Journal of Magnetism and Magnetic Materials 381, 1 (2015); doi: 10.1016/j.jmmm.2015.01.011. Authors include: C. K. Chandrana, J. A. Neal, D. Platts, B. Morgan, and P. Nath (P-21).
The Laboratory Directed Research and Development (LDRD) program funded the work, which supports the Lab’s Global Security mission area and the Science of Signatures science pillar by developing portable flow cytometry technology for the separation of cells. Technical contact: Pulak Nath
Theoretical
Magnetic reconnection: a powerful cosmic particle accelerator
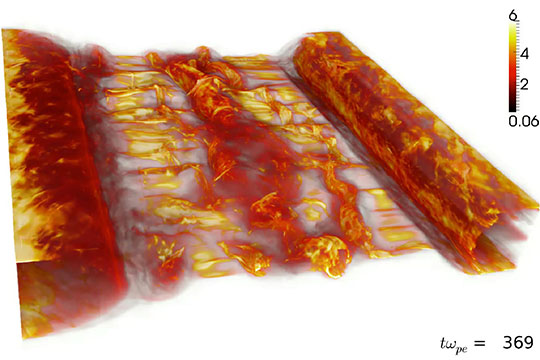
Figure 10. The volume rendering of the current density in the largest 3D simulation. Instabilities in the reconnection layer lead to multiple flux rope structures and turbulent magnetic fields.
The primary mechanism revealed by large 3D kinetic simulations is known as a “Fermi process” in which particles bounce back and forth in magnetic flux ropes. The spectral index can be even harder than that from strong shocks, making it unique in explaining particle acceleration under extreme circumstances. This research indicates that magnetic reconnection is a credible mechanism for the production of cosmic rays and high-energy emissions. NASA’s newly launched magnetospheric multi-scale spacecraft in our local natural laboratory can test many aspects of the theory. The findings may explain the gamma-ray emission from high-energy astrophysical systems such as pulsar wind nebulae and relativistic jets from supermassive black holes detected by the LANL-led High Altitude Water Cherenkov (HAWC) Observatory.
, Fan Guo (Nuclear and Particle Physics, Astrophysics and Cosmology, T-2) and collaborators have conducted two- and three-dimensional (3D) kinetic simulations to investigate relativistic magnetic reconnection and the associated particle acceleration. The team found that magnetic reconnection could be an efficient particle accelerator and may hold the key to explain astrophysical high-energy emissions. The researchers used the vector particle-in-cell (VPIC) code developed by LANL on peta-scale computers to demonstrate that a large fraction of available magnetic energy is converted into a nonthermal power-law particle energy spectrum.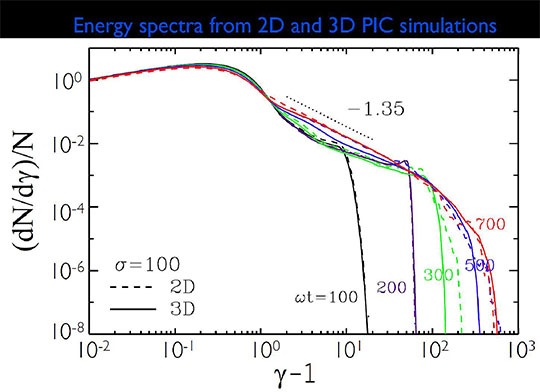
Figure 11. Evolution of particle energy spectra from 2D and 3D simulations. A power-law distribution develops through the nonthermal acceleration in the reconnection layer.
References:
“Particle Acceleration and Plasma Dynamics during Magnetic Reconnection in the Magnetically-dominated Regime,” Astrophysical Journal 806, 167 (2015): doi: 10.1088/0004-637X/806/2/167. Authors include: Fan Guo and Yi-Hsin Liu (T-2), William Daughton (Plasma Theory and Applications, XCP-6), and Hui Li (NASA Goddard Space Flight Center).
“Scaling of Magnetic Reconnection in Relativistic Collisionless Plasmas,” Physical Review Letters 114, 095002 (2015); doi: 10.1103/PhysRevLett.114.095002. Authors include: Yi-Hsin Liu and Fan Guo (T-2), William Daughton (XCP-6), Hui Li and Michael Hesse (NASA Goddard Space Flight Center).
“Formation of Hard Power-laws in the Energetic Particle Spectra Resulting from Relativistic Magnetic Reconnection,” Physical Review Letters, 113, 155005 (2014); doi: 10.1103/PhysRevLett.113.155005. Authors include: Fan Guo (T-2), Hui Li (NASA Goddard Space Flight Center), William Daughton (XCP-6), and Yi-Hsin Liu (T-2).
Different aspects of the work at LANL are funded by the Laboratory Directed Research and Development (LDRD) program and the DOE Office of Science in collaboration with the Center for Magnetic Self Organization (CMSO), and by NASA through the Heliospheric Theory Program. The research is part of the Blue Waters sustained-petascale computing project, which the National Science Foundation and the state of Illinois sponsor. Researchers performed additional simulations at the National Center for Computational Sciences at Oak Ridge National Laboratory and with LANL institutional computing. The research supports the Lab’s Nuclear and Particle Futures science pillar. Technical contact: Fan Guo